Abstract
Wet heatwaves can have more impact on human health than hot dry heatwaves. However, changes in these have received little scientific attention. Using the ECMWF Reanalysis v5 reanalysis dataset, wet-bulb temperatures (Tw) were used to investigate the spatial-temporal variation of wet heatwaves in Eurasia for 1979–2017. Wet heatwaves were defined as three day or longer periods when Tw was above the 90th percentile of the summer distribution and characterized by amplitude, duration and frequency. Maximum values of amplitude, close to 31 °C, occur in the Indus–Ganges plain, the lower Yangtze valley, and the coasts of the Persian Gulf and Red Sea. Significant positive trends in the frequency and amplitude of wet heatwaves have occurred over most of Eurasia though with regional variations. Changes in heatwave amplitude (HWA) are largely driven by changes in summer mean Tw. For Eurasia as a whole, increases in temperature contribute more than six times the impact of changes in relative humidity (RH) to changes in Tw HWA. Changes in Tw have a strong dependence on climatological RH with an increase in RH of 1% causing a Tw increase of 0.2 °C in arid regions, and only increasing Tw by 0.1 °C in humid regions. During Tw heatwaves in Europe, parts of Tibet, India, East Asia and parts of the Arabian Peninsula both temperature and humidity contribute to the increase in Tw, with temperature the dominant driver. During wet heatwaves in part of Russia, changes in humidity are weak and the increase in Tw is mainly caused by an increase in temperature. In the Mediterranean and Central Asia, RH has fallen reducing the increase in Tw from general warming.
Export citation and abstract BibTeX RIS

Original content from this work may be used under the terms of the Creative Commons Attribution 4.0 license. Any further distribution of this work must maintain attribution to the author(s) and the title of the work, journal citation and DOI.
1. Introduction
There is no universal definition of a heatwave, but extreme events associated with particularly hot sustained temperature have significant impacts on human health, infrastructure and biophysical systems (Easterling et al 2000, Welbergen et al 2008, McMichael and Lindgren 2011, Xu et al 2016, Campbell et al 2018). The effects of the most lethal heatwaves are not only due to high temperature, but also to the effects of humidity (Steadman 1979a, 1979b). Hot and humid conditions can be more dangerous than equivalently hot but dry conditions, as the ability of humans to cool themselves by sweating is diminished as relative humidity (RH) increase (Wehner et al 2017).
Eurasia is a region with large population densities, which is vulnerable to environmental extreme events such as heatwaves. Recent examples of heatwaves include the European heatwave of 2003 (Christoph and Gerd 2004) and the Russia heatwave of 2010 (Russo et al 2014), which caused more than 70 000 heat-related death rolls; the 2013 heatwave in eastern China (Hou et al 2014, Xia et al 2016) and the 2017 Shanghai heatwave (Chen et al 2019), causing widespread drought and cascading economic losses (e.g. Xia et al 2018). Many studies have found an increase in dry heatwave occurrences during the last few decades over most of the Eurasia (Karl et al 1995, Meehl and Tebaldi 2004, Alexander et al 2006), whereas others studies point out the regional differences in these trends (Zhai et al 2003, Perkins-Kirkpatrick et al 2016).
Previous research on heatwaves has mainly emphasized changes in temperature with limited studies of recent changes in hot and wet heatwave events in Eurasia. Most previous studies on wet heatwaves used observations from local ground stations that can provide accurate temperature and humidity datasets. For example, using station observation temperature and RH datasets, Ding (2011) classified the wet and dry heatwave by mean RH and investigated the geographical patterns and temporal variation of heatwave events in China. However, there are some issues when using observations directly, such as missing records, inconsistencies through time, especially the RH datasets, which has serious quality issues (Zhu et al 2015, Li et al 2020). Using reanalysis and CMIP5 dataset, Russo (2017) explored global humid heat wave hazards at different levels of global warming and found the magnitude and apparent temperature peak of heatwaves have been amplified by humidity, such as Chicago heatwave in 1995 and China heatwave in 2003. Based on a reanalysis dataset, Kang (2018) calculated the wet bulb temperature (Tw) in the North China Plain, which can be used to measure high temperature and humidity climatic events, finding that heatwave risk had increased due to the anthropogenic effects of irrigation in this region. However, this kind of wet heatwave analysis has not been previously studied in Eurasia.
This study focuses on the spatial-temporal variation of hot and wet extreme events in Eurasia, and the reason for its changes since 1979. We use Tw to identify the wet heatwave. This is a suitable index to investigate the impact of climate change on heat stress (Pal et al 2016, Tong et al 2017). While the core temperature of human body is around 37 °C, the skin temperature is slightly cooler at 35 °C. If the environmental Tw exceeds 35 °C, then human body is unable to dissipate heat by sweating, leading to hyperthermia (Sherwood et al 2010, Tong et al 2017). In the current climate, Tw rarely exceeds 31 °C at daily timescale (Sherwood and Huber 2010). Other combined empirical temperature and humidity indices have been used to investigate the impacts of climate change on heat stress such as wet bulb globe temperature (WBGT) (e.g. ISO 1989) which is the basis for time limitations of work in different heat exposure standards. The black globe temperature is not observed in many places (Luo and Lau 2019), which makes WBGT more complex and difficult to measure. In contrast, Tw can be measured using standard methods and it also provides a physically based relationship to the human body's core temperature (Stull 2011, Pal 2016). Hence, Tw is feasible for daily use and has been used more often in climate studies (e.g. Pal and Eltahir 2016, Raymond et al 2017).
This paper investigates changes in wet heatwave events in Eurasia using Tw, calculated from a reanalysis dataset. In this study, we compare the variation of the temperature and humidity during wet heatwaves in Eurasia, focusing on the large scale pattern of change in wet heatwaves and analyzing the reason for differential regional changes. The data and methods are described in section 2; the results are presented in section 3, and main conclusions and discussion are given in section 4.
2. Data and methods
2.1. Study region and datasets
We defined Eurasia as land points within the following eight (Giorgi and Francisco 2000) regions: Northern Europe (NEU), Mediterranean Basin (MED), Central Asia (CAS), Tibet (TIB), North Asia (NAS), East Asia (EAS), South Asia (SAS) and part of Sahara (SAH), noting that our MED and SAH regions do not include parts of North Africa. The analysis used the ECMWF Reanalysis v5 (ERA5) reanalysis data for the period from 1979 to December 2017 with a spatial resolution of 0.25° × 0.25° (Hersbach et al 2020). This study used daily mean temperature (Tmean) and RH from 1979 to 2017. The time during the day when maximum RH and maximum temperature are not the same (Maqsood et al 2005, Hong and Wang 2009). Tw would be overestimated if maximum temperature and maximum RH were used to calculate it. Thus, in this study, we computed daily Tw from mean temperature and RH. RH in China was homogenized (Freychet et al 2020) by comparison with a nudged global atmospheric model simulation, as China is the only large-scale region where RH observation systems were systematically changed around 2003–2004 (Li et al 2020).
2.2. Quantification of wet heatwaves
Tw was calculated using equation (1) of Stull (2011) giving Tw (°C) as a function of temperature (Tmean) and RH (%)
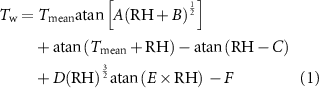
where A, B, C, D, E, F are constants (A = 0.152, B = 8.314, C = 1.676, D = 0.004, E = 0.023, F = 4.686), obtained by fitting functions (Stull 2011).
Extreme Tw days were defined as days when Tw was above the 90th percentile summer (June–August) days. A wet heatwave event was defined as three or more consecutive extreme Tw days during the summer period (Russo et al 2015, Freychet et al 2020).
Several climate indices have been applied in order to quantify the dry heatwave duration (HWD) and intensity based on daily maximum and minimum temperature (Meehl and Tebaldi 2004, Alexander et al 2006, Fischer and Schär 2010, Perkins et al 2012) and there is no one universal index. Based on the previous research, we defined three new indices to represent the frequency, duration, and intensity of wet heatwaves: heatwave day frequency (HWF) which is the total number of days in heatwave events per summer; HWD which is the duration of the longest heatwave in a summer; heatwave amplitude (HWA) which is the maximum Tw value in all heatwave events in a summer.
2.3. Tmean and RH contribution to Tw
Based on equation (1), Tw is affected by Tmean and RH. In order to quantify the contributions of changes in Tmean and RH to changes in Tw, we calculated ∂Tw/∂Tmean (oC/oC) and ∂Tw/∂RH (oC/%) which are:
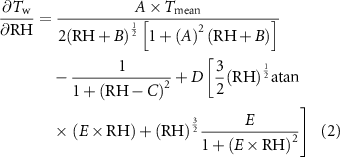

A, B, C, D, E, F are the same as equation (1). For small changes in mean temperature () and RH (
) then the change in wet bulb temperature is (Taylor 1717):

where is a residual representing 2nd, and higher, order terms. We use this to partition changes in wet bulb temperature due to changes in mean temperature and RH.
2.4. Statistical methods
For the regional analysis trends were computed at each grid point and the median computed. Uncertainty in the median was computed by bootstrapping (Efron and Tibshirani 1994) by randomly sampling 1000 times from all the grid points in the area calculating the median value. Unless otherwise stated, we use 5%–95% uncertainty ranges. Significance in grid-point trends was taken from the standard error in the linear regression trend assuming each calendar year is independent.
3. Results
3.1. Climatological characteristics of wet heatwaves in Eurasia
Figures 1(a) and (b) show the annual mean HWF and HWA during the summer (June, July and August) in Eurasia. Wet heatwave frequency is largest (more than five days/summer) in Saudi Arabia, parts of Southern and Eastern Asia (figure 1(a)). For HWA (figure 1(b)), two areas of wet heatwaves are located in SAS and EAS with largest values in the Indus–Ganges plain and in eastern China. Regional mean HWA is 24.4 °C and 22.1 °C for SAS and EAS, respectively. These monsoonal regions have high temperature and humidity, which leads to higher Tw heatwave intensity (figure 1(b)). High values of HWA also occur around the Persian Gulf with HWA values of 30.3 °C. This is the largest value of HWA in Eurasia and is close to the dangerous Tw of 31 °C (Sherwood and Huber 2010), which is rarely exceeded in the current climate. One possible reason for this is the high humidity in coastal areas, which contributes to the high Tw value. Therefore, it is meaningful to investigate the regional differences of the contribution of humidity and temperature on Tw.
Figure 1. Geographical distribution of the mean value of (a) HWF (unit: days), (b) HWA (unit: °C) for summers during 1979–2017. The color bar in (b) is non-uniform to focus on high values. (c) Box and whisker plot of the liner trend of summer HWF (red boxes), HWD (green boxes), and HWA (blue boxes) in eight Giorgi regions (Giorgi and Francisco 2000) during 1979–2017 from the 0.25 × 0.25 ERA-5 dataset. For each boxplot, the bottom and top of the boxes are the lower and upper quartiles, respectively, the bar near the middle of the box is the median, and the whiskers show the 5% and the 95% values.
Download figure:
Standard image High-resolution imageSAS (figure 1(c)) is the region with the highest significant (p < 0.1) increasing trends in regional mean HWF and HWD, with 87% and 80% of the grids, respectively, showing an increasing trend indicating that heatwaves have become more frequent and last longer here. In most regions, HWA shows significant (p < 0.1) positive trends with largest values in NEU and MED, at 0.38 °C and 0.32 °C·decade−1, respectively. However, the CAS region shows little change in wet heatwave indicators.
3.2. Changes of humidity and temperature during heatwave days and summer mean
Tw is affected by both temperature and humidity and so the contributions of temperature and humidity to wet heatwave changes might have strong regional differences in Eurasia. To explore this we first compare the mean value and the linear trend of temperature and humidity during 1979–2017, then the quantitative contributions of Tmean and RH to Tw changes are discussed in section 3.3.
When a heatwave occurs, largest Tw values occur in the monsoon regions including SAS and EAS (figure 2(a)). Highest Tmean values, during wet heatwaves, occur mostly in part of SAH, especially Saudi Arabia, CAS, SAS and EAS. High RH centers are located in SAS, EAS, and part of NAS. Lower RH in Saudi Arabia, CAS and Mongolia was due to high temperatures and low humidity in these regions. In contrast, in northeast NAS, low values of temperature lead to high RH in this region.
Figure 2. Geographical distribution of the mean value of (a), (b) Tw, (c), (d) Tmean, (e), (f) RH during heatwave days (left) and mean value on heatwave days minus summer mean (right) during 1979–2017. To calculate the heatwave day mean minus summer mean, for each grid point and each year, we calculate the mean value of Tw, Tmean and RH for all heatwave days and then subtract the mean value of summer Tw, Tmean and RH for that year. The color bars in (a) and (c) are non-uniform to focus on high values.
Download figure:
Standard image High-resolution imageTo examine how different heatwave conditions are from summer-mean conditions, we calculated the differences of mean Tw, Tmean, and RH on heatwave days from the 1979 to 2017 summer mean (figures 2(b), (d), (f)). Where these differences are large, this is where heatwave Tw is larger than the summer mean. Tw differences between wet heatwave and summer days increased smoothly from South to North (figure 2(b)) with largest differences in Arctic Ocean coast. This suggests that wet heatwaves are more extreme in these regions, which might lead to more serious impact. Tmean (figure 2(d)) differences are similar to Tw differences and are also smooth, possibly indicating the dominant contribution (see below) of dry-bulb temperature to Tw. This implies that wet heatwaves are different from conventionally defined dry heatwaves, because dry heatwaves often happens under high pressure centers (anticyclone) hence with dry air (Baldi et al 2006, Gershunov et al 2009). For RH differences (figure 2(f)), high values are found at SAS, TIB and north EAS. In most regions, heatwave RH is about 20% higher than the climatological mean, though with significant regional heterogeneity, suggesting a large, though heterogeneous, increase in RH when wet heatwaves occur.
To understand the regional trends in Tmean and RH when wet heatwave happens, we analyze trends in Tw, Tmean and RH during heatwaves (figures 3(a), (c), (e)). In NEU, northwest EAS, and parts of Arabia and TIB, increasing Tw is accompanied by an increase in temperature and RH. This implies that increasing Tw in these regions is due to increases in both temperature and humidity. In eastern parts of CAS and SAS, as Tw increases, a significant positive trend in RH is observed, with no significant positive Tmean trends in these areas. An extreme case is in parts of SAS, where Tmean declined significantly in heatwave days in part of the region. Thus, the increases in Tw in these regions are likely mainly due to the increase in RH. In western Russia, southeastern EAS and the northern Mediterranean, where Tw increased significantly, Tmean has significant positive trends and changes in RH are not significant. Thus, Tw increases are likely being driven by Tmean increases. Quantitative analysis of the contributions of changes in Tmean and RH to changes in Tw is shown in section 3.3.
Figure 3. Geographical distribution of the linear trend of (a), (b) Tw, (c), (d) Tmean, (e), (f) RH during heatwaves (left) and heatwave mean minus summer mean (right) during 1979–2017. Shading indicates non-significant (p < 0.1) trends. To calculate the trend of heatwave mean minus summer mean, for each grid and each year, we calculate the mean value of Tw, Tmean and RH for heatwave days and subtract the mean value of summer Tw, Tmean and RH, and then calculate trend and significance from the difference time series.
Download figure:
Standard image High-resolution imageOver most of Eurasia, differences between the trends of heatwave day and summer mean for Tw, Tmean and RH are not significant during 1979–2017 (figures 3(b), (d), (e)). This suggests that significant changes of Tw, Tmean and RH in heatwave days are largely due to changes in the summer mean.
3.3. Quantitative contribution from changing Tmean and RH to Tw
To measure quantitatively the sensitivity of Tw to Tmean and RH, respectively, we calculated ∂Tw/∂Tmean (oC/oC) and ∂Tw/∂RH (oC/%) from mean conditions during wet heatwaves (equations (2) and (3)). For most of Eurasia, an increase of 1 °C in Tmean during wet heatwaves increases Tw by 0.6 °C–1 °C (figure 4(a)) with lowest sensitivity in arid regions and highest sensitivity in Scotland, Ireland, Southeast Asia, parts of India and the Pacific coast of Russia. A 1% increase in RH causes a Tw increase of 0.05 °C–0.2 °C (figure 4(b)) with higher RH sensitivity in the Arabian Peninsula, eastern China, South EAS and SAS and lower sensitivity in Scandinavia, Siberia and TIB.
Figure 4. Geographical distribution of the (a) ∂Tw/∂Tmean (100*°C/oC; equation (2)) and (b) ∂Tw/∂RH (100*°C/%; equation (3)) during heatwave days (right) for 1979–2017, where Tw, RH, Tmean are the mean values for all heatwave days during 1979–2017. (c) Plot of median trendT w (gray boxes), trendT mean*∂Tw/∂Tmean (red boxes), calculated by equation (2) and the median of the trendRH*∂Tw/∂RH (blue boxes), calculated by equation (3), residual = trendT w− (trendT mean*∂Tw/∂Tmean + trendRH*∂Tw/∂RH) (purple boxes), in eight sub-regions during 1979–2017. For each region, the short black horizontal line shows the median of all the grids in this region; the vertical bar shows the small uncertainty interval of the median. The 5% and 95% of the medians show the uncertainty interval. The color bars in (a) and (b) are non-uniform to make the distribution clear. Geographical distribution of (d) trend Tmean*∂Tw/∂Tmean and the (e) trend RH*∂Tw/∂RH during 1979–2017. Shading indicates non-significant (p < 0.1) trends. Color bar in (d) is three times than in (e).
Download figure:
Standard image High-resolution imageTo measure quantitatively the contributions of changes in Tmean and RH to changes in Tw (see equation (4)), both the sensitivity of Tw and the changes of Tmean and RH were considered (figures 4(c)–(e)). Tmean is the largest driver of changes in Tw though the quantitative contribution depends on both the sensitivity of Tw to temperature and the change in temperature (figures 4(c)–(e)). Considering Eurasia as a whole, changes in Tw are largely driven by changes in temperature, but the contributions of temperature and humidity to the HWA changes exhibit significant regional differences (figure 4(c)). Over the eight regions, the dominant driver of increasing median Tw on heatwave days is dry-bulb temperature with only small contributions from RH trends. In CAS drying offsets the warming effect leading to only small changes in Tw. The Mediterranean region also shows a drying effect which reduces the temperature driven increase in Tw. In contrast, the TIB region shows a modest increase in Tw over the dominant temperature driven increase from changes in RH.
4. Discussion and conclusion
In summary, this work provides an overview of the changes in summer wet heatwave in Eurasia and investigates the reasons for its changes. Significant increasing trends in the frequency, duration and intensity were observed in most of Eurasia, especially the monsoon regions including SAS and EAS, which show the highest HWA during 1979–2017. In most of Eurasia, Tw shows a significant increasing trend during heatwave days. The main driver of this change is increasing dry-bulb temperatures with reductions in RH partly offsetting this increase in some regions. The decrease trend of RH in these regions suggests that the saturated water vapor pressure increases faster than actual water vapor pressure with rising temperature, consistent with Lou and Lau (2019). They use apparent temperature as a measure of heat stress, and, like us, find heat stress in China is mainly caused by high temperature, rather than high RH. Significant changes in Tw during wet heatwave days are largely due to the Tmean changes in summer mean. Compared with humid areas, arid areas are more sensitive to changes in RH and less sensitive to Tmean increases. This result is partly consistent with the conclusion of Wang et al (2019), who used Chinese observations. Wang et al (2019) also show that humidity has higher dominance in the arid and semiarid regions compared with in the Southern and Northeastern China. In our study, Eurasia is divided into eight sub-regions which highlights the regional difference. Over the eight regions, the dominant driver of increasing median Tw on heatwave days is dry-bulb temperature, whereas changes in RH play a relatively minor role. This is different in some sub-regions. In CAS drying offsets the warming effect leading to only small changes in Tw. The Mediterranean region also shows a drying effect which reduces the temperature driven increase in Tw. In contrast, the TIB region shows a modest increase in Tw over the dominant temperature driven increase from changes in RH.
These results are derived from a reanalysis gridded dataset which may introduce errors through interpolation of inhomogeneous station observations. This is especially so for RH observations (Willett et al 2008, 2014, Freychet et al 2020), which are prone to inhomogeneous biases due to non-climate changes in the local observing system. For example, Song (2012) found that RH in most of China showed a reducing trend. Some researchers have suggested that changes in the Chinese RH observing system from manual to automated is the main reason for the decline of RH in humid areas during the early 2000s (Yu and Mou 2008, Yuan et al 2010) likely due to use of Soviet practice and instruments for manual wet-bulb measurements in humid regions (Hu 2004). In this work, we used ERA5 RH data corrected in China as Freychet (2020), which partly reduced the impact of inhomogeneity of RH in this region, leading to an increasing trend of Tw in China. Therefore, it is important to explore the sensitivity of these results to use of other higher quality global reanalysis data. In addition, the main findings of this study are dependent on the definition of heat stress parameter. In this study, wet heatwave is measured by Tw. However, many other heat stress indicators, such as heat index (Rothfusz and Headquarters 1990, Lou and Lau 2019), humidex (Masterson and Richardson 1979), discomfort index (Epstein and Moran 2006), temperature exposure (Jones et al 2015), etc, have also been used in the previous research. Although many of them describe the joint effects of high temperature and humidity, a thoroughly and unified assessment is needed to reduce uncertainty in the future.
Overall, it is important to recognize regional differences in setting heatwave adaptation policies to reduce the impacts of wet heatwaves. Our main finding is that summer-mean temperature changes are the main driver for changes in wet HWA in Eurasia. Thus, one can hypothesize, with global warming, such increases in wet heatwave events will continue into future leading to more severe society impacts especially dealing with human health. Based on two greenhouse gas scenarios, Pal et al (2016) suggests that by the end of the century (2071–2100), annual Tw in parts of southwest Asia (e.g. Dhabi, Dubai) was projected to exceed 35 °C, the threshold which people cannot exposure in for more than 6 h, several times in the 30 years. However, current projections of Tw were mainly based on the variation in the mean Tw, and the research region was usually small and not suitable for identifying the regional differences across a large scale. More research is needed to understand the impacts of extreme wet heat events now and in the future, and different mitigation measures should be considered in different regions.
Acknowledgments
S Y and Z Y were supported by the Strategic Priority Research Program of Chinese Academy of Sciences (Grant No. XDA20020201) and the Ministry of Science and Technology international collaboration (Grant No. 2017YFE0133600). S Y carried out this work as a visiting Student to the University of Edinburgh funded through the UK-China Joint Research and Innovation Partnership Fund PhD. Placement Programme 20180252701. S F B T and N F were supported by the UK-China Research and Innovation Partnership Fund through the Met Office Climate Science for Service Partnership (CSSP) China as part of the Newton Fund.
Data availability statement
The data that support the findings of this study are available upon reasonable request from the authors.