Abstract
We assess the influence of humans on burned area simulated with a dynamic global vegetation model. The human impact in the model is based on population density and cropland fraction, which were identified as important drivers of burned area in analyses of global datasets, and are commonly used in global models. After an evaluation of the sensitivity to these two variables we extend the model by including an additional effect of the cropland fraction on the fire duration. The general pattern of human influence is similar in both model versions: the strongest human impact is found in regions with intermediate productivity, where fire occurrence is not limited by fuel load or climatic conditions. Human effects in the model increases burned area in the tropics, while in temperate regions burned area is reduced. While the population density is similar on average for the tropical and temperate regions, the cropland fraction is higher in temperate regions, and leads to a strong suppression of fire. The model shows a low human impact in the boreal region, where both population density and cropland fraction is very low and the climatic conditions, as well as the vegetation productivity limit fire. Previous studies attributed a decrease in fire activity found in global charcoal datasets to human activity. This is confirmed by our simulations, which only show a decrease in burned area when the human influence on fire is accounted for, and not with only natural effects on fires. We assess how the vegetation–fire feedback influences the results, by comparing simulations with dynamic vegetation biogeography to simulations with prescribed vegetation. The vegetation–fire feedback increases the human impact on burned area by 10% for present day conditions. These results emphasize that projections of burned area need to account for the interactions between fire, climate, vegetation and humans.
Export citation and abstract BibTeX RIS

Original content from this work may be used under the terms of the Creative Commons Attribution 3.0 licence.
Any further distribution of this work must maintain attribution to the author(s) and the title of the work, journal citation and DOI.
1. Introduction
Fire appeared on Earth around 400 million years before the present day (Scott 2000), and shaped the evolution of ecosystems and plant traits (Pausas and Schwilk 2012). Humans started to use fire approximately 1–1.4 million years ago, which was an important step in the evolution of human technology (Berna et al 2012, Gowlett et al 1981). Habitual use in Europe followed 300–400 thousand years ago (Roebroeks and Villa 2011). This tight connection and long evolution of fire, vegetation and humans hampers the separation between natural and anthropogenic fire regimes. Here, we use a global modeling approach to separate human influences on burned area from natural fire occurrence.
Humans influence various aspects of fire regimes, including number of fires, fire size (Hantson et al 2015), burned area, intensity, emissions or seasonality (Magi et al 2012). Humans influence the area burned directly, by igniting and suppressing fires, but also indirectly by modifying the vegetation structure and composition, and by fragmenting the landscape (Bowman et al 2011). These direct and indirect effects can either promote or suppress fire. Conversion of forest to grasslands can lead to a higher flammability of the landscape, increasing fire occurrence (Cochrane 2003). In contrast, a reduction or limitation of fire spread due to human action is expected due to harvesting or built infrastructure.
Satellite data provide detailed information on fire regimes, and reveal a strong human influence across the globe (Archibald et al 2013). The spatial patterns suggest a decrease in burned area with increasing population density (Bistinas et al 2014, Knorr et al 2014) or cropland fraction (Bistinas et al 2014, Andela and van der Werf 2014). Human influence was also detected in a trend analysis showing a strong decrease in burned area over the last two decades (Andela et al 2017).
The global charcoal database provides information on the variability in fire activity over longer time scales. In these charcoal records, variations which oppose the trend expected due to climatic conditions are interpreted as human driven (Marlon et al 2008). The increase in charcoal records from 1750–1870 is attributed to human influence, linked to population growth and land-use changes, and a subsequent decrease, due to intensification of land use. The trends in the charcoal record are confirmed by CO ice core records for the Southern Hemisphere (Wang et al 2010).
The human influence on fire regimes is also observed locally: analysis of single charcoal records in New Zealand shows a strong change in fire regime, with an increase in fire occurrence, after the arrival of humans (McWethy et al 2010). In the tropics, land use change is known to influence the fire regime, and has potential to modify large parts of this important biome (Cochrane 1999). The higher flammability of deforested areas leads to more frequent fire occurrence, and can further damage the surrounding forested areas and amplify the influence of fires set by humans on the vegetation and fire regime (Cochrane 1999).
In this study, we assess the influence of humans on fire occurrence in terms of burned area in a global fire enabled dynamic vegetation model JSBACH–SPITFIRE. We first evaluate the model along the human dimensions included in the model, which are population density and the cropland fraction, and extend the model with a stronger influence of the cropland fraction. We then investigate where burned area is strongly influenced by humans. We further assess whether the feedback between vegetation and fire amplifies the human impact. We discuss limitations and uncertainties of our approach, and compare our results to available literature.
Table 1. List of simulations with settings for the computation of ignitions, fire duration, land use and vegetation dynamics.
Reduced fire duration due to | |||||
---|---|---|---|---|---|
Simulation | Human ignitions | Population density | Crops | Land use | Vegetation dynamics |
HumanDynVeg | yes | yes | no | yes | yes |
HumanDynVegCrops | yes | yes | yes | yes | yes |
NaturalDynVeg | no | no | no | no | yes |
NaturalLUDynVeg | no | no | no | yes | yes |
HumanFixVeg | yes | yes | no | yes | no |
NaturalLUFixVeg | no | no | no | yes | no |
2. Model description and simulation setup
2.1. JSBACH–SPITFIRE
JSBACH (Raddatz et al 2007, Brovkin et al 2009) is the land surface model of the MPI Earth system model (MPI-ESM) (Giorgetta et al 2013). We use the JSBACH version with the soil carbon model YASSO (Goll et al 2015). The process-based fire model SPITFIRE (Thonicke et al 2010) has been evaluated for present day burned area and carbon emissions (Lasslop et al 2014), and is described in detail and in comparison to other global fire models in Rabin et al (2017). JSBACH simulates the terrestrial carbon and water cycle in a process-based way. It provides fuel amounts, vegetation composition and soil moisture for the fire model. The fire model reduces the carbon pools according to the simulated combustion of biomass, and computes tree mortality due to fire. Fire can start if ignition (lightning or human) occurs and a sufficient amount of fuel leads to sufficiently high fire line intensity. Based on the fuel size and moisture, the combustion completeness and rate of fire spread is computed. The combination of the rate of spread with the fire duration (see below) yields the burned area. Tree mortality is a function of the fire line intensity and the residence time of the fire. SPITFIRE accounts for the human influence based on population density and the cropland fraction. Population density (PD [inhabitants km−2]) is used to compute the human ignitions (nh,ig)):

The factor 0.4 was adjusted to tune the model to reproduce the global burned area of the GFED3 dataset (Giglio et al 2010). The regionally varying factor (a (Nd)) was introduced to reflect regional and cultural differences in the human use of fire as a tool. As the burned area fraction varies globally by several orders of magnitude, the factor (which varies between 0.11 and 0.33) has rather low influence. This was found in a study using ORCHIDEE–SPITFIRE (Yue et al 2014) and for JSBACH–SPITFIRE simulations without vegetation dynamics, where the spatial variability of ignitions has low influence on the spatial patterns of burned fraction (Lasslop et al 2014). The JSBACH–SPITFIRE model reduces the fire duration (DF in minutes) with increasing population density, to improve the relationship between population density and fire size (Hantson et al 2015). The maximum fire duration was increased from 4 hours in the original model version (Thonicke et al 2010) to 12 hours (Hantson et al 2015), assuming that most fires cease during night. While fires in reality often continue during night, due to the low variation of ignitions in the model, a new fire will start the next day unless the conditions for a fire change (changes in moisture conditions or fuel load), and therefore reduce the influence of the 12 hours threshold on longer term fire occurrence. DF is computed in three different ways: (1) as a function of the fire danger index (FDI):
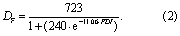
(2) using PD and the FDI:
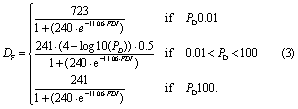
and (3) additionally reducing DF with increasing cropland fraction (fcrop) by multiplying equation (3) with (1–fcrop). The first approach is used when no human influence is included, the second includes human influences, and the third is an extension to improve the model's sensitivity to the cropland fraction (see Appendix).
Land use is included in JSBACH following the protocol of Hurtt et al (2011), and described in detail in Reick et al (2013). In SPITFIRE, fires are excluded from cropland area, and pastures are treated the same as natural grasslands. Moreover, land use influences fire by changing the carbon stocks of the available fuels (Wilkenskjeld et al 2014).
In this study we use JSBACH in the offline mode, forced with meteorological forcing which was extracted from simulations with the MPI-ESM version 1.1 for the historical period 1850–2005. The spatial resolution is 1.875° × 1.875°, and the temporal resolution is 30 min. The fire routine is called once a day. For the spinup the first 28 years of forcing (1850–1877) were recycled, and CO2 concentration fixed at the value of 1850 (284.725 ppm). The historical simulation from 1850 to 2005 uses transient climate, CO2 concentration and land use. The population dataset is based on (Klein Goldewijk 2001). The decadal temporal resolution is interpolated to annual values, and updated at the beginning of the year.
2.2. Simulations
To assess the impact of humans on the fire regime, we compare burned area from simulations with (simulation name includes tag 'human') and without human influence (tagged 'natural'). We include two different ways of accounting for the human impact on fire duration. The first decreases the fire duration with population density; the second additionally reduces fire duration with increases in the cropland fraction (tag 'Crops'). As the representation of human ignitions in the model already includes a suppression of ignitions for high population density, the simulations cannot factor out fire suppression by humans—only the combined effect of human enhancement and suppression of fires can be separated. A decrease in burned area when including human effects, however, indicates that human suppression dominates, while an increase in burned area would indicate that the additional ignitions are more important. We assess the importance of the feedback between fire and vegetation on the influence of humans on the fire regime, by comparing simulations with (DynVeg) and without (FixVeg) dynamic vegetation. In that case, a consistent initial vegetation cover was necessary, and land use needed to be included (NaturalLU simulations). Therefore, only the amplification of vegetation dynamics on the direct human influence on ignitions and fire suppression could be assessed. The effect of fire–vegetation feedback on the human impact was quantified by comparing the difference between HumanDynVeg and NaturalLUDynVeg to the difference between HumanFixVeg and NaturalLUFixVeg simulations. Overall six simulations with different settings in the computation of ignitions, fire duration, land use and vegetation dynamics were performed (table 1). We performed a spinup simulation of 1000 years for the simulations with dynamic natural vegetation. The spinup period with fixed vegetation cover was only 300 years, as only the rather fast litter carbon pools need to be in equilibrium to stabilize the fire regime.
Data analysis and plotting was done using R 3.3.3 (R Core Team 2016). In the regional analysis we define the boreal region as all grid cells with latitudes >60°, the temperate region with latitudes between −30° and −60° or 30° and 60°, and the tropical with latitudes between −30° and 30°.
Figure 1. Global distribution of differences in burned fraction with and without human influence. Based on a comparison of the HumanDynVeg and NaturalDynVeg simulation (a) and on a comparison between HumanDynVegCrops and NaturalDynVeg (b) for present day (average over the years 1996–2005).
Download figure:
Standard image High-resolution imageFigure 2. Burned fraction along a gradient of NPP for tropical (a), temperate (b) and boreal regions (c) for the present day (average over the years 1996–2005). Lines were fitted using generalized additive models, shaded areas indicate the 95% confidence interval of the mean. Please note the different axis scales of the subplots.
Download figure:
Standard image High-resolution image3. Results
3.1. Human impact on burned area
3.1.1. Global impact and spatial patterns
We assess the influence of humans on burned area by comparing the simulation HumanDynVeg (includes human set fires, fire suppression as a function of population density and land use) or simulation HumanDynVegCrops (additionally including suppression as a function of the cropland fraction) with the simulation NaturalDynVeg (only lightning ignitions and no human influence). Globally, we can find regions with increased and reduced burned area due to human influence for the present day (figure 1). The spatial pattern of the effect of humans is similar for both simulations including the human effects: HumanDynVeg and HumanDynVegCrops. We find a strong suppression of burned area in regions with high population density and high cropland fraction (Eastern part of the USA and Europe, India). Overall, we find suppression of fire mostly in the temperate regions. Tropical regions (except India) mainly show strong increases in burned area due to the human impact (figures 1 and 2(a)), indicating that the influence of higher ignitions is strong there, and fire is limited by ignitions. The correlation between the differences in burned area and human ignitions in the simulations HumanDynVeg and NaturalDynVeg is low (R2 = 0.1), but highly significant (p-value <0.001). The low correlation between human ignitions and change in burned area due to human effects indicates that the effect of increases in ignitions is strongly modulated by other factors, such as climate and vegetation.
From pre-industrial to present day (over the period from 1870 to 2005), burned area decreases by 19.6% (HumanDynVeg) and 23.6% (HumanDynVegCrops) if human influences are accounted for (table 2). In contrast, the simulation considering only natural effects shows a 5.4% increase in burned area.
Table 2. Global burned area [Mha] for preindustrial (average over 1860–79) and present day (average over 1996–2005) for all simulations.
Simulation | Burned area preindustrial | Burned area present day |
---|---|---|
HumanDynVeg | 449.21 | 361.16 |
HumanDynVegCrops | 413.20 | 315.61 |
NaturalDynVeg | 289.76 | 305.42 |
NaturalLUDynVeg | 228.26 | 222.84 |
HumanFixVeg | 449.86 | 366.36 |
NaturalLUFixVeg | 279.46 | 242.65 |
3.1.2. Human impact on burned area along gradients of plant productivity
The human impact on burned area along gradients of plant productivity is similar for the simulations HumanDynVeg and HumanDynVegCrops. The additional suppression in simulation HymanDynVegCrops leads to a lower burned area. The effect of humans is generally low at low NPP (< 40 g C m−2 year−1)(figure 2). Burned area in regions with low productivity is limited by the fuel buildup (Krawchuk and Moritz 2011, Bistinas et al 2014). In the tropical region both simulations with human influence show an increase in burned area for intermediate NPP, compared to the simulation without human influence; for low and high NPP the difference is small (figure 2(a)). Moreover, the human impact shifts the maximum of burned area towards higher productivity in the tropical regions. While burned area is limited by the available fuel for low productivity areas, climatic conditions are increasingly limiting for high NPP.
In temperate regions, the influence of humans reduces the burned area at intermediate to high NPP values (40–110 g Cm−2 year−1) (figure 2(b)). Population density is on average similar for tropical and temperate regions; cropland fraction is, however, 50% higher in temperate regions, leading to a reduction in burned area in contrast to the higher burned area in the tropics. Another potential explanation is the difference between modeled human and lightning ignitions: human ignitions are assumed constant within the year, while lightning ignitions include a seasonality. In temperate regions, the lightning strikes are most frequent in summer, the season with high fire risk. In the tropics the number of lightning strikes is highest in the rainy season, where fire occurrence is limited by meteorological conditions. This may lead to an ignition limitation in the tropics during the dry season.
For the boreal regions, no significant differences (95% confidence intervals of the fitted lines overlap) are simulated (figure 2(c)). In the boreal regions NPP is rather low (below 74 g Cm−2 year−1), burned area there is, however, not only limited due to the low productivity, but also by shorter periods with high fire risk. Low population density in the boreal region (factor 100 lower compared to temperate and tropical regions) and smaller cropland fraction (0.003 on average, compared to 0.1 in the tropics and 0.15 in temperate regions) additionally explain the small difference between the simulations with and without human impact.
3.2. Amplification of human impacts through vegetation dynamics
Fire leads to a reduction in woody vegetation and an increase in highly flammable herbacious vegetation, forming a positive feedback. Due to this positive feedback alternative stable states under the same climatic conditions occur in JSBACH–SPITFIRE (Lasslop et al 2016). This means that increases in burned area can lead to the crossing of a tipping point driving the system in a stable low-tree-cover state with higher burned area. The effect of humans on fire is therefore expected to be higher in simulations with dynamic vegetation cover. The effect is quantified by comparing the difference between the HumanDynVeg and NaturalLUDynVeg simulations to that between HumanFix and NaturalLUFix. Both increases and decreases due to the human impact are stronger if vegetation cover is dynamic (figure 3). When vegetation cover is fixed, the human impact increases the burned area for preindustrial from 287.16 (NaturalLUFix) to 454.83 (HumanFix) Mha (58%), while when including the feedback between fire and vegetation, the human impact increases the burned area from 235.46 (NaturalLUDynVeg) to 456.61 (HumanDynVeg) Mha (93%). If the vegetation–fire feedback is excluded the differences in global burned area caused by the human impact are reduced by 24% in the pre-industrial period and 10% for present day (table 2, (HumanFix-NaturalLUFix) divided by (HumanDynVeg-NaturalLUDynVeg). The lower effect for present day might be due to the larger areas controlled by human land use.
Figure 3. Human impact for present day with dynamic vegetation (HumanDynVeg-NaturalLUDynVeg) and fixed vegetation (HumanFix-NaturalLUFix).
Download figure:
Standard image High-resolution image4. Discussion
4.1. Uncertainties in modeling the human impact on fire
Over the last century, technological advances in fire fighting and increased resources spent on fire management have certainly impacted the human capacity to suppress fires. Humans change the local vegetation in many ways that may influence the fire regime—for instance, the introduction of new species with higher flammability can increase the area burned, or land management leading to a fuel reduction through harvest or grazing can lead to a reduction of burned area (Bowman et al 2011). These effects, especially their variation over time, are not represented in the model. The limited generalizable understanding and lack of global information limit the possibility of reflecting this knowledge in global models. The representation of humans in global models is largely based on data analyses of spatial datasets, which show that human parameters (e.g. population density and land use) do explain part of the variation observed for burned area, and how they influence burned area.
Modeling human ignitions and suppression as a function of population density is common in global fire models (Hantson et al 2016). It is often assumed that for low population density ignitions increase, and that for high population densities fires are increasingly suppressed. Some models include the suppression for high population density in the function of the ignitions, others include this influence only or additionally on the rate of spread or fire duration, and some models include the human suppression in a scaling function that reduces burned area directly (Hantson et al 2016, Rabin et al 2017). The suppression of fire for high population density is, however, now common in global fire models (Hantson et al 2016, Rabin et al 2017). This spread in the representation of human impact in models reflects the versatile results of data analysis. On the global scale, based on multivariate analysis of global spatial datasets, an increase in burned area with increases in population density even for very low population densities was not detected (Bistinas et al 2013), or detected only with high uncertainty (Knorr et al 2014, Lasslop et al 2015). In a study for Africa, an increase for low population density was found for the number of fires, but not for the burned area (Archibald et al 2009). When applying statistical models to smaller regions in the United States, response functions with a distinct maximum—similar to the modeling approach used here—were detected for many regions, but the exact shape varied widely (Parisien et al 2016).
In the evaluation of the modeled burned fraction along increasing population density, we found an underestimation of burned fraction for high and low population densities (figure A1). However, these regions with high underestimation only contribute 2.5% to the global burned area. Occurrence of ignitions in the model is based on the lightning and population datasets, as humans might also set fires in uninhabited places, or lightning strikes might not be tracked. A small background ignition rate might help to overcome this model error. Due to the small contribution of these areas to the total burned area, we expect that this can only have a small influence on the spatial patterns of human impact. The evaluation (figure A1) does not give a clear indication that our approach of increasing ignitions for low population densities and then reducing the same after a certain threshold should be replaced by a function that represents only suppression of humans, as indicated by some global data analysis (Bistinas et al 2013, Knorr et al 2014).
The second human dimension in global fire models is the fraction of croplands. Global data analysis confirms that the effect of croplands is to reduce burned area (Bistinas et al 2014, Andela and van der Werf 2014). Croplands are often simply excluded from burning in fire models (Rabin et al 2017). LPJ–LMfire is an exception, and includes a passive fire suppression assuming that the cropland fraction is a good proxy for landscape fragmentation (Pfeiffer et al 2013). Our extended model version, which reduces fire duration with increasing cropland fraction, improves the model performance (figure A1) but does not have a strong influence on the patterns of human influence on burned area.
Overall, our model-based study thus shows a plausible picture of limited influence of humans on burned area in regions with low vegetation productivity or meteorological conditions limiting burned area. The strong suppression of humans due to dense population and croplands in temperate regions is well supported by literature, and expected for other global fire models due to a similar representation of the human impact. The effects of humans in sparsely populated areas are most uncertain based on available literature, and comparing global model structures (e.g. whether they allow for anthropogenic enhancement of burned area). We find enhancement of burned area due to the human influence mostly in the tropics, due to the lower cropland fraction (on average cropland fraction is 50% higher in the temperate regions). A lower suppression for the tropical regions compared to the temperate regions can therefore also be expected for models not allowing for an enhancement of burned area due to human ignitions.
4.2. Comparison with previous studies on the human effect on fire
Besides the satellite data analyses discussed in the previous section, paleo-data contain information on the variability of fire. These datasets are especially valuable as they cover long time periods. On the other hand, the uncertainty of the datasets is larger, and it is less clear which aspect of the fire regime is captured (Brücher et al 2014). Variability in the charcoal record could be the result of variations in the area burned, or variations in the biomass consumed—for instance, due to changes in vegetation composition. Charcoal (Marlon et al 2008) and ice core (Wang et al 2010) data indicate a strong decrease in biomass burning between the pre-industrial era and present day. This decrease has been attributed to human action, as the decrease in fire occurrence is associated with a strong increase in population density, while the climate records cannot explain a decrease in fire occurrence. Our results confirm these previous findings, showing a strong decrease in burned area only, when human effects are included.
Previous studies have posed hypotheses about the effect of humans on fire regimes based on charcoal data analysis and theoretical considerations (McWethy et al 2013, McWethy et al 2010). They suggest that in temperate regions the influence of humans amplifies fire in regions with high net primary productivity (NPP), while for intermediate NPP suppression is expected (McWethy et al 2013). Our model results show the suppression for intermediate NPP, which disappears for higher NPP, but do not show an increase of burned area due to human influence for high NPP. Paleo records indicate that the arrival of humans is often followed by a strong increase in fire occurrence (McWethy et al 2010). Although the model approach does not distinguish between initial and later phases of human settlement, the cropland fraction might be an indicator for this development. In present day temperate regions, humans have already passed this initial phase, and the region is now mainly characterized by fire suppression.
In tropical regions, a study investigating the effect of different burning patterns in tropical savannas did not find effects on the total area burned (Van Wilgen et al 2004). However, increases are expected, especially if the disturbance of primary forests leads to a higher flammability of the landscape (Cochrane 1999). Based on the uncertainties in how to model the effect of humans for low population densities, and the variety of results from data analysis (see above), we consider the very strong enhancement in the tropics as most uncertain.
5. Conclusions
We assess the impact of humans on the global wildfire distribution, based on a global vegetation model, and compare the results to previous studies. The model accounts for human influence based on population density and land use, which are commonly used human parameters in global fire models. The model reproduces the sensitivity of burned area to both variables, in comparison to satellite datasets. This study shows that including the human dimension in global fire modelling is crucial, and confirms the human driven decrease since preindustrial times observed in the global charcoal dataset. We find that the human impact is low for the boreal region, strongly suppresses fire in the temperate regions and enhances fire in the tropical regions. The difference between temperate and tropical regions is likely due to higher land use in the temperate regions, while the low impact in boreal regions can be explained by much lower population density and cropland fraction. We find the strongest human influence in regions with intermediate productivity, where burned area is strongly limited neither by fuel availability nor by climatic conditions. We show that the interaction between fire and vegetation dynamics amplifies the human impact. This feedback will be important not only when investigating the human impact on fire, but also in other studies investigating changes in fire regimes or changes in vegetation in fire affected areas. Projections of fire occurrence need to understand and account for the interactions between fire, climate, vegetation and humans.
Appendix: Evaluation of the response of burned area to the human dimensions
To evaluate the impact of humans on the simulated fire occurrence, we compare the simulated burned area to burned area derived from satellite data for the present day, along a gradient of the human dimensions of the model; these are population density and cropland fraction (figure A1). We use the burned area datasets of the GFED database version 3 (Giglio et al 2010), 4 (Giglio et al 2013) and 4s (Randerson et al 2012).
Figure A1. Average annual burned area along a gradient of the logarithmic population density (left) and cropland fraction (right) for present day (average over 1996–2005 for the model simulations and 1997–2006 for the GFED burned area datasets (version 3, 4 and 4s), population density is representative for the year 2000, cropland fraction is the model mean over 1996–2005). Lines were fitted using generalized additive models; shaded areas indicate the 95% confidence interval of the mean.
Download figure:
Standard image High-resolution imageThe simulations including the human impact show a similar variation of burned area for different population densities compared to the GFED burned area datasets. The GFED4s dataset shows higher maximum values for the burned fraction than GFED3 and GFED4. GFED4s includes small fires that are usually not captured. These small fires are mostly burned croplands. Cropland burning is not included in JSBACH–SPITFIRE; the comparability of the model is therefore higher with GFED3 and GFED4. The model reproduces the maximum value of the GFED3 and 4 dataset well, but underestimates fire occurrence for low and high population density. The simulation without human effects on the fire regime shows a maximum for higher population densities compared to the observations; the simulation underestimates burned area for low population densities and overestimates burned area for high population densities.
The evaluation along the cropland fraction shows an overestimation of burned fraction for high cropland fraction if the model simply excludes croplands from burning. Including croplands in the computation of the fire duration (simulation HumanDynVegCrops) improves the mismatch for high cropland fraction. The simulation without human effects mainly increases the burned area with increasing cropland fraction, and is not able to reproduce the observed pattern with a maximum for a cropland fraction of around 0.05.
The comparison between the simulations including and excluding human effects confirms that both human effects—humans as an additional ignition source, and the suppression by humans of fire spread, are important in reproducing the variation of burned area along gradients of human influence. Natural fire regimes would be shifted towards regions with stronger human influence. The spatial correlation with the GFED4 dataset increases from 0.05 for the NaturalDynVeg simulation to 0.38 for both simulations including the human impact.
Another benchmark for the human influence on burned area was recently provided by Andela et al (2017). Satellite data show a negative trend in global burned area over the last 18 years, which was attributed to the human influence (Andela et al 2017). As our simulations only cover the period until the year 2005, we recomputed the trend over the years 1997–2006 for GFED versions 3 and 4. To account for the uncertainty due to the high interannual variability, we computed the trend ten times, each time excluding one year. We find negative trends for GFED version 3 (−2.6% to −1.2% per year), mixed trends for GFED version 4 (−0.5% to 0.6% per year), negative trends for the HumanDynVeg simulation (−0.6% to −0.02%), and mixed trends for the HumanDynVegCrops simulation (−0.5% – 0.1% per year). This metric is therefore not sufficiently robust over this short time period to clearly indicate whether the human influence is sufficiently strong in the model.
Acknowledgments
We thank K Naudts for helpful discussion and comments on the manuscript. We thank two anonymous reviewers and Sandy Harrison for constructive reviews of the manuscript. Data and analysis scripts can be obtained from publications@mpimet.mpg.de. We would like to thank the DKRZ for the excellent computing facilities and the GFED team for the burned area datasets.