Abstract
Reciprocal asymmetric transmission (AT) phenomenon has received much attention due to various polarization applications, however, high-efficiency AT still remains out of reach in the optical range. In order to improve AT of linearly polarized light, low-loss dielectric metamaterial with twisted resonators should be designed and investigated. The stereometamaterial is constructed by an array of bilayer 90° twisted H-shaped Ge dimers. Based on the transmission matrix, numerical simulation and multipolar resonances analysis, the polarization responses in all-dielectric stereometamaterial are characterized. The simulated results show that the AT parameter reaches up to 98% around 194 THz. In addition, the AT can be engineered via geometrical parameters. The proposed stereometamaterial also exhibits strong circular dichroism that is direction-independent. The multipole decomposition calculations can be utilized to understand the resonant mechanism.
Export citation and abstract BibTeX RIS
1. Introduction
As an important candidate, anisotropic and chiral metamaterials have realized various polarization manipulations such as negative refraction, circular dichroism (CD) and asymmetric transmission (AT) [1, 2]. The AT phenomenon is one of the most important effects in chiral metamaterials, indicating a total intensity difference between two counter-propagating directions of electromagnetic waves. The AT effect is reciprocal and can be understood as polarization conversion dichroism [3]. Since the AT effect of circularly polarized wave was firstly reported by Fedotov et al [3], the AT phenomena have been extensively studied in various chiral metamaterials [4–13]. Single layer metamaterials always exhibited week AT effect from microwave to optical ranges [3–7]. In order to enhance the AT effect of the circularly polarized wave, several methods have been studied such as multilayer metamaterials [8–11] and dielectric metamaterials [12, 13]. Meanwhile, the AT effect of linearly polarized light has received much attention in recent years [14–25]. A criterion based on the transmission matrix was reported for only achieving asymmetrical transmission for linearly polarized wave [14]. A 3D low-symmetry metamaterial with single wire and L-shaped resonators has been proposed to experimentally and theoretically verify the AT phenomenon of the linearly polarized light with 25% intensity difference at the wavelength of ∼1200 nm [15]. In most cases, the AT effects have been realized in metamaterials consisting of bilayer identical resonators with a twist angle [16–25]. The interlayer coupling of two orthogonal resonators, electromagnetic wave tunneling and Fabry–Perot cavity are helpful to enhance the polarization conversion and the AT effect [17–22]. Metamaterials with AT will be promising candidates to manipulate the polarization state of light in optical systems and offer a way to realize compact polarization converter and on-chip directional photonics devices. However, efficiencies of the AT effects in the optical frequency hardly exceed 60% due to high radiation and metallic losses [15, 23–25]. Achieving high-efficiency AT phenomenon is still challenging in the optical range.
Recently, all-dielectric metamaterials have also attracted much attention due to their advantages such as low loss and the coexistence of strong electric and magnetic resonances [26–28]. In all-dielectric metasurfaces, high refractive index materials are always exploited such as Si, Ge, GaAs and GaP [28]. A variety of terahertz and photonic devices based on all-dielectric metamaterial have been studied such as polarization manipulation [12, 29], beam deflector [30], hologram [31], structural color [32], optical nonlinearity [33], absorber [34] and magnetic mirror [35]. For circularly polarized light, single-layered all-dielectric metasurfaces have been proposed to realize 76% AT parameter [36] and giant tri-band spin-selective AT [37]. The AT effects of linearly polarized waves have been also reported in all-dielectric metamaterials, operating in the terahertz and optical ranges [38, 39]. In bilayer metamaterials with two twisted resonators, several materials were studied how their relative permittivities affected the AT phenomenon. The metamaterial with Ge resonators exhibited more pronounced AT phenomenon with about 90% AT parameter. In fact, the AT effects of linearly polarized wave have often been studied in stereometamaterials that refer to metamaterials with the same constituents but different spatial arrangements [40]. However, high-efficiency AT in stereometamaterial still remains out of reach in the optical range and meanwhile the AT phenomenon of linearly polarized light should be further studied.
In order to achieve high-efficiency AT of linearly polarized light, all-dielectric stereometamaterial with twisted resonators will be investigated. The metamaterial consists of an array of bilayer 90° twisted H-shaped Ge dimers. One polarization passes through the stereometamaterial in terms of polarization conversion while the other polarization is completely suppressed along the same propagation direction, leading to a pronounced AT phenomenon. Based on the transmission matrix, numerical simulation and multipolar resonances analysis, the polarization responses in all-dielectric stereometamaterial are characterized. The simulated results show that the AT parameter reaches up to 98% around 194 THz. In addition, the AT can be designed via geometrical parameters. The proposed stereometamaterial also exhibits strong CD that is direction-independent. The electromagnetic field distributions can be utilized to understand the resonant mechanism. The proposed all-dielectric stereometamaterial provides a promising way to realize polarization manipulation of electromagnetic waves and polarization information detection.
2. Theoretical method
Here, a theoretical description of electromagnetic wave transmission passing through an optical element is given in a three-dimensional Cartesian coordinate system. When the plane wave is normally incident upon the metamaterial along the forward direction (i.e. the −z direction), the Jones matrix (complex amplitude matrix) provides a simple way to represent the transmission relationship [15, 41]. The formula is given below
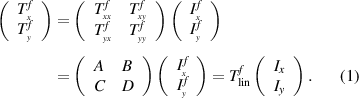
Here Ix, Iy represents the complex amplitudes of incident x- and y-polarized waves, respectively. The superscripts which are denoted as f and b indicate the forward and backward propagation directions of incident wave. The four elements of A, B, C, D can be simply used to replace Tij . Since the consideration is limited in a reciprocal optical system, the transmission matrix of the metamaterial along the backward propagation direction can be expressed as below [41]

The Λ matrix can describe the basis change from the linear base to the circular base, which is given as follows

The transmission matrix of circularly polarized light can be given using equation (4). The forward matrix of the reciprocal metamaterial can be written using equation (5).


Here, left-handed circularly polarized light (LCP) and right-handed circularly polarized light (RCP) are denoted by '−' and '+', respectively. As known, the AT phenomena are caused by polarization conversion dichroism and can be discussed according to polarization conversion efficiency along different propagation directions [3, 15]. The symbol Δ denotes the AT parameter that can be evaluated via the strength of polarization conversion. In the case of linearly polarized light, Δ is given by equation (6), in which the subscripts x or y indicate the polarization state of incident light.

Therefore, the relationship between the off-diagonal elements in the transmission matrix defines the AT effect as long as the co-polarization transmission coefficients are kept unchanged in the reversed propagation direction.
3. Results and discussions
The H-shaped and U-shaped resonators have widely studied to realize various phenomena and applications such as stereometamaterials [40], gradient-index metasurface [42], coplanar imaging [43], hyperbolic spoof plasmonic metasurfaces [44] and polarization convertor [45]. The concept of stereometamaterial offers an important degree of freedom to investigate the interplay of electric and magnetic interactions as well as alternative potential for manipulating optical polarization [40]. Stereometamaterials refer to metamaterials with the same resonators but different spatial orientations. Here, we proposed an all-dielectric H-shaped stereometamaterial. The unit cell is an H-shaped dimer with a SiO2 spacer layer, in which the bilayer patterns are the same but there is a twist of 90° between them, as depicted in [40]. Figure 1(a) depicts the schematic diagram of the proposed stereometamaterial. While linearly polarized light is incident along the −z axis, the stereometamaterial reveals an obvious AT phenomenon. The polarization conversion is allowed from x to y polarization along the forward propagation direction while x to y polarization conversion is forbidden along the backward propagation direction. Figure 1(b) shows the structural view of the unit cell. The period p of the square lattice is 1200 nm. The thicknesses of Ge and the spacer layer SiO2 are tg = 150 nm and td = 250 nm, respectively. The other geometrical parameters are chosen as below, l1 = 300 nm, l2 = 900 nm, w = 175 nm, s = 262.5 nm. The parameter s describes the shift with respect to the symmetry geometry. The H-shaped dimer here lacks the mirror line along the x axis. The H-shaped dimer has a twist angle of 90° around the z direction. The relative permittivity and tangent loss of Ge resonator are 18 and 0.001, respectively [39]. The relative permittivity of the spacer SiO2 layer is 1.9. The commercial software CST Microwave Studio 2016 was used to simulate the transmission of the stereometamaterial by use of both transient and frequency solvers. The periodic boundary conditions are used along the x and y directions representing an infinite structure. The S-parameter convergence criterion has been satisfied after using adaptive tetrahedral mesh refinement. The final mesh typically contained about 100 000 tetrahedrons. Assuming λ = 1500 nm, the minimum and maximum edge lengths of 5 nm (∼0.0033λ) and 150 nm (∼0.1λ), respectively.
Figure 1. (a) The schematic diagram of the proposed stereometamaterial showing the AT effect of linearly polarized light. (b) The geometry of the unit cell. The geometrical parameters are given below, td = 250 nm, tg = 150 nm, l1= 300 nm, l2= 900 nm, w= 175 nm, p = 1200 nm, s= 262.5 nm. The H-shaped dimer has a twist angle of 90° around the z axis.
Download figure:
Standard image High-resolution imageHere we define the transmission amplitude as . The transmission properties of the stereometamaterial along the forward and backward propagation directions are illustrated in figure 2. Due to the orthogonal arrangement of two identical H-shaped resonators, the co-polarization transmission curves along the forward and backward propagation directions exactly coincide with each other regardless of x- or y- polarized light in figures 2(a) and (b). There is an obvious difference between two cross-polarization transmission coefficients txy
and tyx
in the whole frequency range, as shown in figure 2(a). Although the transmission curves of txy
and tyx
are different below 160 THz, their resonances are very similar and the cross-polarization transmission peaks correspond to the co-polarization transmission dips in figure 2(b). When the frequency is higher than 160 THz, the difference between two cross-polarization coefficients rapidly increase. The maximum difference occurs at about 194 THz when the peak resonance of tyx
and the dip resonance of txy
nearly have the same resonant frequency. Importantly, the peak resonance of tyx
is nearly close to 100% while three other transmission coefficients are completely suppressed to zero. When the propagation direction is reversed, the transmission curves of txy
and tyx
exchange with each other. Thus the stereometamaterial exhibits high-efficiency AT phenomenon.
Figure 2. (a) The transmission spectra of the stereometamaterial along the forward propagation direction (the −z axis). (b) The transmission spectra of the stereometamaterial along the backward propagation direction (the +z axis). The co-polarization transmission curves of both x- and y-polarized waves coincide with each other.
Download figure:
Standard image High-resolution imageThe AT parameter spectra of the stereometamaterial along the forward propagation direction (the −z axis) is displayed in figure 3(a). The AT parameter of x-polarized wave is contrary to that of y-polarized wave. The polarization rotation angle and the ellipticity angle are given as follows:
Figure 3. (a) The AT parameter spectra of the stereometamaterial along the forward propagation direction (the −z axis). (b) The polarization azimuth and ellipticity spectra of the stereometamaterial along the forward propagation direction (the −z axis).
Download figure:
Standard image High-resolution image

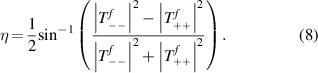
Figure 3(b) reveals the polarization azimuth and ellipticity spectra of the stereometamaterial along the forward propagation direction (the −z axis). The polarization azimuth spectra of the stereometamaterial at normal incidence shows that the polarization rotation within the frequency range of 180–194 THz is intensive.
In order to further know about polarization properties of the all dielectric H-shaped stereometamaterial, it is necessary to discuss the dependence of the AT effect on angles of incidence with respect to z axis. Figure 4 shows angular dependence of the AT parameter when the incidence angle α of x-polarized light varies from 0 to 80°. It can be seen intuitively from figure 4(a) that the metamaterial exhibits the strongest AT effect at normal incidence. In other words, the AT parameter decreases significantly as the incidence angle increases. Figure 4(b) describes the AT spectra of x-polarized light when the incident angle α is 0°, 30° and 60°, respectively. When the incidence angle α is changed only in the vicinity of normal incidence, the AT phenomenon in the stereometamaterial maintains its high efficiency around 194 THz.
Figure 4. (a) Dependence of the AT parameter on a function of the incidence angle α. (b) Curves of the AT parameter Δx when α is 0°, 30° and 60°.
Download figure:
Standard image High-resolution imageNext, the dependence of the AT parameter on the geometry will be investigated in detail. When the shift s varies, the simulated result of the AT parameter for incident x-polarized light is shown in figure 5(a), in which the other geometrical parameters are kept unchanged. Obviously, the shift s is very important for determining the presence or absence of the AT phenomenon. Around the optimal value of the shift s, the AT parameter reaches up to 98% at about 194 THz. In our proposed stereometamaterial, the AT phenomenon can properly be engineered via the shift s. Apparently, when s slowly increases from 0 nm, x-polarized light undergoes the AT effect at around 150 THz. As s further increases, the high-efficiency AT effect can be found in the high frequency range. The maximum AT effect occurs around 194 THz. The AT parameter of y-polarized light is exactly opposite to that of x-polarized light. Figure 5(b) shows the AT parameter for x-polarized light when s is 242.5, 262.5 and 302.5 nm.
Figure 5. (a) Dependence of the AT parameter of incident x-polarized light as a function of the shift s. The other geometrical parameters are kept unchanged as aforementioned. (b) Curves of the AT parameter Δx when s is 242.5, 262.5 and 302.5 nm. (c) Dependence of the AT parameter of incident x-polarized light as a function of the length l1. (d) Curves of the AT parameter Δx when l1 is 220, 250 and 300 nm, marked by three lines in panel (c).
Download figure:
Standard image High-resolution imageFigure 5(c) illustrates the dependence of the AT parameter on the length l1 for incident x-polarized light while the other geometrical parameters remain unchanged. Obviously, the AT effect is highly efficient within relative large range of l1. According to the evolution of the AT parameter, the polarization conversion of x-polarized light slowly decreases with increasing l1. The AT spectra of x-polarized light are shown in figure 5(d) for several fixed l1. The larger l1 leads to the weaker AT phenomenon. Especially, the AT effect suddenly decreases when l1 exceeds 300 nm.
The dependence of the AT parameter on the width w is studied in figure 6. The maximum AT effect is marked by the circle dot in figure 6(a). It is apparent that when the width w increases from 115 to 195 nm, the polarization conversion and AT of linearly polarized light is red-shifted and the high-frequency pass band becomes narrow. Figure 6(b) describes the AT spectra of x-polarized light when the width w is 135, 165 and 175 nm, respectively. When the width w is changed in a wide range, the high-frequency AT phenomenon maintain its high efficiency.
Figure 6. (a) Dependence of the AT parameter of incident x-polarized light as a function of the width w. (b) Curves of the AT parameter Δx when w is 135, 165 and 175 nm, marked by three lines in panel (a). The black dot denotes the maximum of the AT phenomenon.
Download figure:
Standard image High-resolution imageThe thickness of the spacer layer affects the coupling effect between the front resonator and the back resonator. Figure 7(a) shows the simulated AT spectra in the all-dielectric stereometamaterial as a function of the thickness td of the spacer layer SiO2. The high efficient AT phenomenon will change with varying the thickness td . It is found that the strongest polarization conversion is blue-shifted in the considered frequency band as the thickness of the SiO2 spacer layer increases. When the thickness of the spacer layer exceeds 210 nm, the designed stereometamaterial exhibits a red-shifted AT phenomenon of incident x-polarized light when the beam is normally incident. Figure 7(b) indicates AT spectra for three different td . It is important to know the change of high frequency AT phenomenon. Both the low- and high-frequency AT effects are relatively weak when td is 150 nm. As td increases, the high frequency AT peak increases until it approaches 98%.
Figure 7. (a) Dependence of the AT parameter of incident x-polarized light as a function of the thickness td of the space layer. (b) Curves of the AT parameter Δx when td is 150, 260 and 300 nm, marked by three lines in panel (a). The black dot denotes the maximum of the AT phenomenon.
Download figure:
Standard image High-resolution imageThe proposed all-dielectric stereometamaterial differently responses to RCP and LCP waves as shown in figure 8. Here the CD is defined as equation (9).
Figure 8. (a) The transmission spectra of the all-dielectric stereometamaterial for incident circularly polarized light. (b) CD spectrum of the proposed all-dielectric stereometamaterial.
Download figure:
Standard image High-resolution imageFigure 8(a) shows transmission spectra of circularly polarized light according to equation (9) when the light propagates along −z axis. The transmission curves of t−− and t++ are quite different, but the polarization conversion curves of the t−+ and t+− are completely identical, indicating the absence of the circular AT phenomenon. According to figure 8(b), the positive maximum of CD is about 0.61 and the negative maximum of CD is about −0.68. Clearly, the simulated result shows that the stereometamaterial with linear AT effect will exhibit strong CD response for sensing surrounding medium.

In order to understand the mechanism of the AT phenomenon and polarization conversion, Z-component magnetic field distributions in the x–y plane are presented in figure 9, corresponding to three resonant frequencies of 141 THz, 156.4 THz and 194 THz, respectively. Clearly, there are circular displacement currents around the H-shaped resonators in both the top and bottom layers at low resonant frequencies of 141 THz and 156.4 THz, leading to an enhanced Z-component magnetic field distribution at the center of the unit cell of each layers as shown in figures 9(b), (c), (e) and (f). At 141 THz, the two Z-component magnetic field distributions of the top and bottom H-shaped resonators are in-phase while they are out-of-phase at 156.4 THz. As shown in figures 9(a) and (d), the Z-component magnetic field distributions of the all-dielectric stereometamaterial at 194.1 THz are entirely different from two low-frequency resonances. Firstly, the strongest Z-component magnetic field distributions locate inside the interior of H-shaped rods instead of the center distribution of the unit cell at two low-frequency resonances. Secondly, there are nodes along the H-shaped rods that are time-varying movement along the H-shaped rods. Thirdly, the time-varying twist senses of Z-component magnetic field distributions are the same, therefore Z-component magnetic fields of the top and bottom H-shaped resonators are always in-phase. Figures 9(g)–(i) show magnetic field vector distributions of the all-dielectric H-shaped stereometamaterial at three resonant frequencies of 141 THz, 156.4 THz and 194 THz in the y–z plane.
Figure 9. (a)–(f) Z-component magnetic field distributions of the all-dielectric H-shaped stereometamaterial for incident x-polarized wave at three resonant frequencies of 141 THz, 156.4 THz and 194 THz in the x–y plane. (a)–(c) the top layer and (d)–(f) the bottom layer. (g)–(i) Magnetic field vector distributions of the all-dielectric H-shaped stereometamaterial at three resonant frequencies of 141 THz, 156.4 THz and 194 THz in the y–z plane.
Download figure:
Standard image High-resolution imageIn order to fully recognize the contributions of multiple resonances, the radiating power was calculated to identify types of resonances in the proposed H-shaped stereometamaterial [37, 46–48]. The decomposition calculations indicate how electric dipole (ED), magnetic dipole (MD), toroidal dipole (TD), electric quadrupole (EQ), and magnetic quadrupole (MQ) contribute to the scattering property of the proposed metasurface. For each type of multipole, the far-field scattering is characterized by electromagnetic radiation reemitted by the entire array of the corresponding multipoles. The multipolar decomposition of the microscopic charge-current excitations, within the unit cell of the metamaterial, can be directly linked to far-field macroscopic metamaterial response [46–48]. To quantitatively evaluate the contributions to the electromagnetic scattering of the multipoles, the normalized calculation results in figure 10 indicate that ED, MD, EQ, MQ and TD are main resonances. The decomposition calculations are beneficial to understand what resonances contribute to the radiating power of the proposed H-shaped metasurface. The MD plays the dominant role at 141 THz while the ED and other attribute weekly to the radiating power. The EQ is much stronger than others at 156.4 THz. Different from two low frequency resonances, the resonant AT at 194 THz results from the excitation and overlapping of ED, MD, EQ, MQ and TD. Here the TD, MQ and MD are more dominant, which is similar to the phenomenon in [37]. The multipolar decomposition is important to understand the principle of the AT in all-dielectric stereometamaterial.
Figure 10. Calculated results of multipolar decomposition of scattering spectra in the all-dielectric H-shaped stereometamaterial. ED: electric dipole, MD: magnetic dipole, EQ: electric quadrupole, MQ: magnetic quadrupole, TD: toroidal dipole.
Download figure:
Standard image High-resolution image4. Conclusions
We numerically studied the AT phenomenon in all-dielectric stereometamaterial with twisted H-shaped Ge resonators. The metamaterial consists of an array of bilayer 90° twisted H-shaped Ge dimers. One polarization passes through the stereometamaterial in terms of polarization conversion while the other polarization is completely suppressed along the same propagation direction, leading to a pronounced AT phenomenon. The transmission matrix, numerical simulation and multipolar resonances analysis are characterized. The simulated results show that the AT parameter reaches up to 98% around 194 THz. In addition, the AT can be designed via structural parameters. The proposed stereometamaterial also exhibits strong CD that is direction-independent. The electromagnetic field distributions can be utilized to understand the resonant mechanism. The proposed all-dielectric stereometamaterial provides a promising way to realize polarization manipulation of electromagnetic waves and polarization information detection.
Acknowledgments
This work was supported by the National Natural Science Foundation of China (NSFC) under Grant Nos. U1931121, 91750107 and 61675054, partly by the Natural Science Foundation of Heilongjiang Province in China under Grant Nos. ZD2020F002 and ZD2018015, by 111 project to the Harbin Engineering University under Grant No. B13015, by the Fundamental Research Funds for the Central Universities under Grant Nos. 3072020CFT2501 and 3072020CFT2528 and by the Postdoctoral Scientific Research Developmental Fund of Heilongjiang Province under Grant Nos. LBH-Q9097.