Abstract
Urban runoff and wastewater/sewage input are majorly responsible for the contamination of urban streams. In streams where wastewater input is not a considerable input, the importance of urban runoff as a mechanism of contaminant transport and delivery from urban surfaces to receiving waters is even more apparent. Extensive studies on two such streams in Southern Ontario, Canada yielded data on the occurrence and levels of multiple contaminant groups (polycyclic aromatic hydrocarbons and quinones, benzotriazoles (BTs), BT ultraviolet stabilizers, organophosphate esters, herbicides) and the influence of factors such as temperature, rainfall characteristics, and land use. Here, we collectively examined the data from these studies to identify any trends and further insights. Using concentration-discharge relationships, we found that the transport dynamics of many particle-bound compounds are strikingly similar to each other, and to that of suspended solids in which they were quantified, suggesting a single, predominant source. Similar urban to rural ratios across compound groups and strong correlations with road density further support the existence of a dominant source and point to traffic as this source, respectively. Although road traffic had not previously been implicated as a major source of many of the investigated compound groups, their uses suggest that traffic-related sources are very plausible. Overall, this work highlights that traffic is a major source of a surprisingly wide array of organic contaminants to urban surfaces, and subsequently to nearby streams.
Export citation and abstract BibTeX RIS

Original content from this work may be used under the terms of the Creative Commons Attribution 4.0 license. Any further distribution of this work must maintain attribution to the author(s) and the title of the work, journal citation and DOI.
1. Introduction
Known as the 'urban stream syndrome', the degradation of streams draining urban land results from a combination of elevated contaminant concentrations and altered channel morphology which characterize urban aquatic environments. The delivery of contaminants to these streams follows various mechanisms, some of which include wastewater/sewage input, stormwater runoff, atmospheric washout and legacy contamination [1]. Contaminants found in household and personal care products such as flame retardants, plasticizers, pharmaceuticals, alkylphenols and ultraviolet (UV) stabilizers are commonly delivered to surface water via wastewater inputs [2, 3]. Stormwater runoff is more associated with non-point contaminant sources and can be responsible for the transport of diverse groups of contaminants including nutrients, bacteria, metals, microplastics, and organic contaminants to streams during runoff events [4–7]. Legacy contamination and wet and dry atmospheric deposition, though less important, have also been associated with urban stream contamination for metals and organic pollutants [8–11].
Although wastewater is generally regarded as an important driver of the urban stream syndrome, the impact of stormwater runoff on urban stream degradation is nonetheless important. This is increasingly evident in studies of streams that receive no wastewater input. In a study of storm-induced contamination in a non-wastewater receiving stream in Washington State, USA, contaminants including rubber-derived compounds, benzotriazoles (BTs), corrosion inhibitors, pesticides, and pharmaceuticals were detected at concentrations greater than 500 ng l−1 during a storm, similar to reported concentrations for municipal wastewater effluent [12]. Masoner et al [6] similarly found concentrations of pesticides and polycyclic aromatic hydrocarbons (PAHs) that were higher in urban stormwater than in wastewater effluent and positively correlated with impervious surfaces. In a study in North Carolina, USA, other water quality determinants (e.g. biological oxygen demand, turbidity, total phosphorus, and total suspended solids (SSs)) were found to be significantly correlated with the total rainfall amount indicating mobilization of these pollutants from several non-point sources during precipitation [13]. Contaminated stormwater is highly undesirable, as it can episodically deliver high contaminant concentrations to streams within a short period, posing risks to aquatic biota [14, 15], and also hinders the harvesting and reuse of stormwater as an alternative water source [16].
Of the many non-point contaminant sources, traffic is a major driver of stormwater pollution. Traffic-related pollutants such as road surface wear, tire wear particles (TWPs), brake pad linings, vehicle exhaust, and their associated chemicals have been extensively detected in stormwater [15, 17–19]. Whereas contaminants like PAHs and metals are directly linked to traffic sources via exhaust and brake parts, the link is not so evident for other contaminants. In this paper, we analyze data from earlier urban stormwater investigations with a view to observe common trends and possibly find direct and indirect links to traffic as a source of varied contaminants to surface water.
2. Methods
We relied on previously published data on the concentrations of SSs, 12 PAHs [20, 21], two polycyclic aromatic quinones (PAQs) [21], six BT-UVs stabilizers [22, 23], three BTs [24], four herbicides [25], and nine organophosphate esters (OPEs) [26] in water samples collected during three rainfall events at three sites (headwaters, HWs; middle reaches, MDs; and outlet, OL) in each of two watersheds in Toronto, Canada. While similar in most regards, Mimico Creek and Little Rouge Creek watersheds represent widely different degrees of urbanization. Mimico Creek watershed is densely populated, with high impervious surface coverage, a stream bed partially channelized with concrete, and numerous potential emission sources. Rouge, on the other hand, is draining a largely rural landscape. The rainfall events varied in terms of rainfall amount, antecedent dry period, ambient temperature, and runoff ratios (table 1). Previous publications provide more details on the watersheds, event characteristics, sampling design, and chemical analyses [20–25]. Whereas data for individual contaminant groups have been previously interpreted [20–25], we analyze them collectively here for the first time. In particular, we compared and contrasted the different contaminant groups with respect to (a) concentration-discharge relationships, (b) ratios in the flow-weighted average concentrations (FWACs) between the urban and the rural watershed, and (c) the relationship between those concentrations and road density.
Table 1. Characteristics of sampled rainfall events.
Target compounds | Site | Rainfall (mm) | Two-week antecedent rainfall (mm) | Average runoff ratio (%) | Average air temperature (°C) | |
---|---|---|---|---|---|---|
November 2014 | PAHs, BT-UVs, BTs, herbicides | Mimico | 19.4 | 2.8 | 81 | 6.7 |
Rouge | 18.8 | 2.4 | 45 | 6.2 | ||
October 2015 | PAHs, BT-UVs, BTs, herbicides | Mimico | 37.4 | 10.8 | 63 | 11.8 |
Rouge | 42.2 | 26.2 | 19 | 11.4 | ||
August 2018 | PAHs, BT-UVs, PAQs, OPEs | Mimico | 25.6 | 50 | 25 | 22.8 |
Rouge | 38.4 | 32.3 | 23 | 21.8 |
2.1. Concentration vs discharge (CQ) relationships
Plots of concentration (C) vs discharge (Q) are commonly used to show the relationship between a target analyte and stream discharge over the course of a stormflow event. The patterns generated can provide insight into the source strength and transport pathways of target contaminants to a catchment [21]. CQ plots were generated for particle-bound contaminant groups by (a) normalizing concentration and discharge values to the maximum value for a particular storm using:

where xi represents C or Q at a given timestep during a particular storm event, and xmax and xmin represent the maximum and minimum C or Q values for that storm; and (b) plotting normalized C against normalized Q values. Each relationship is based on between 11 and 16 samples taken during a runoff event, generally representing samples from base flow conditions immediately before the event to base flow after the event had passed.
2.2. Comparing flow weighted average concentrations between urban and rural watersheds
For each event, flow weighted average concentrations (FWAC) for the contaminants were calculated using:
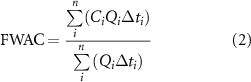
where i and n represent the ith to last samples for a given storm, Ci is the concentration of the ith sample, Qi is the stream discharge at the time of the ith sample, and Δti is the time interval over which Ci and Qi are considered to apply. Particle phase concentrations were averaged for those compound groups that were predominantly sorbed to particles (PAHs, BT-UVs, and PAQs) and dissolved phase concentrations were averaged for those that were mostly dissolved (BTs and herbicides). For OPEs it was done separately for both phases. For each individual contaminant, we then divided the FWAC of a site in Mimico by the FWAC of its paired site in Rouge (i.e. MHW/RHW, MMD/RMD, and MOL/ROL). After averaging these three ratios for all the compounds belonging to a group (PAHs, BT-UVs, PAQs, BTs, herbicides, dissolved OPEs, particulate OPEs), they were then further aggregated across space (average of the three site pairs) and across time (average of the applicable number of events).
2.3. Relating flow weighted average concentrations with road density
Using data sets compiled by DMTI Spatial Inc. and based on road classifications provided by provincial and municipal authorities, ArcGIS was applied to calculate the density of major roads and highways in each sub-watershed [24]. These values were then compared with the total FWACs for each contaminant group at each sub-watershed for each event to assess whether there is a relationship between the intensity of vehicular traffic in a watershed and the observed contaminant concentrations.
3. Results
3.1. CQ relationships for particle-bound contaminants
Figure 1 shows all CQ relationships for SS, and particle-bound PAHs, BT-UVs, OPEs and PAQs. The generation of these plots is explained in the methods section above. It is immediately apparent from figure 1 that all organic compound groups show very similar CQ relationships at most of the sites and during all three events. Furthermore, the CQ relationships for the particles themselves follow the same pattern. Most of the relationships show a clockwise hysteresis, which indicates a CQ ratio that is consistently greater on the rising than on the falling limb of the hydrograph [27], a pattern typically associated with rapid mobilization of contaminants that are close to the sampling point. There are a few obvious exceptions from this overall trend, notably the CQ plots for the outlet of Mimico Creek (MOL) in 2014 and 2015 when the CQ patterns for PAHs (2014 and 2015) and BT-UVs (2014) deviated from that of the SSs.
Figure 1. Concentration discharge patterns for particulate PAHs, BT-UVs, OPEs, PAQs and SSs across sites (columns) and events (rows). Plotted concentration and discharge values are normalized to the maximum value for an event. Data for site MHW during the 2015 event are not sufficiently temporally resolved for inclusion in this plot. OPEs and PAQs were not analyzed during the 2014 and 2015 events.
Download figure:
Standard image High-resolution image3.2. Urban/rural watershed ratios using FWACs
FWACs of dissolved phase BTs and particle-phase PAHs, PAQs, and BT-UVs were consistently ∼20 times higher in the urban Mimico Creek watershed than in the rural Rouge watershed (figure 2). Dissolved and particle-bound OPEs show much lower ratios, indicating either increased atmospheric dispersion between the watersheds and/or the presence of multiple sources of these compounds. Some OPEs are somewhat persistent and subject to atmospheric transport [28], which may account for a less steep concentration gradient, leading to lower urban/rural ratios. The herbicides are the only group that shows urban/rural ratios less than one (0.8) [25].
Figure 2. Box-and-whisker plot of the ratios of the FWACs of different compounds in an urban and a rural watershed (n is the number of individual ratios). The data are grouped by compound group and by phase (dissolved vs particulate). The black squares designate the arithmetic mean.
Download figure:
Standard image High-resolution image3.3. Relationship of FWACs with the density of major roads
Significant relationships were observed between the density of major roads upstream of each sub-watershed and the flow weighted average concentrations for each compound group (figure 3). These relationships were positive for PAHs, BT-UVs, BTs, and PAQs, but negative for the herbicides. The relationships for particulate and dissolved OPEs were not significant.
Figure 3. Relationships between the density of major roads upstream of each sub-watershed and FWACs of different compound groups measured at sites within these sub-watersheds. All relationships are statistically significant except for that of particulate OPEs. Dissolved OPEs are not included in this plot, as the R2 value for this relationship was less than 0.4.
Download figure:
Standard image High-resolution image4. Discussion
Collectively, the remarkable consistency of the CQ plots for SS, and particle-bound PAHs, PAQ, BT-UVs, and OPEs in figure 1 suggests that there is a single source of rapidly mobilized particles that is responsible for delivering all these organic compound groups to the streams. The deviations observed at MOL in 2014 and 2015 are not as prominent in 2018, indicating that the event characteristics in 2014 and 2015 may be the factor responsible (table 1). In figure 2, the narrow range of the urban/rural watershed FWAC ratios (18–25) for most of these same compound groups (PAHs, PAQs, BT-UVs) and additionally for the dissolved phase BTs further suggests that they all have sources related to an activity that is significantly more intense in urban Mimico than in Rouge. Finally, the strong relationship between the FWACs of PAHs, PAQs, BT-UVs, and BTs in a sub-watershed with the road density in that sub-watershed indicates that this activity is plausibly related to road traffic (figure 3). In the case of the particle-phase OPEs, the CQ plots are similar, but the urban/rural ratios are smaller and the relationships with road density are not significant, suggestive of either higher atmospheric mobility or more diverse sources of this group of contaminants. OPEs are widely used as flame retardants and plasticizers, as well as in lubricants, hydraulic fluids, textiles, and lacquers [29, 30], and are generally mixed in as additives and not chemically bonded [31], increasing the possibility of their migration into the environment via multiple routes. The completely divergent behavior of the herbicides when compared to the other compounds, with urban/rural ratios below 1 and negative correlations with road density is not surprising, given their predominant use in agricultural environments [25].
These striking similarities in the source characteristics and the transport and mobilization behavior of compound groups as divergent as combustion byproducts (PAHs and PAQs), atmospheric oxidation products (PAQs), corrosion inhibitors (BTs), UV-stabilizers (BT-UVs), and—to a certain extent—flame retardants (OPEs) may at first seem unusual, and the connection with road traffic is neither entirely obvious. However, a closer look into their individual sources, uses, and applications indeed implicates road traffic as a plausible common source:
- PAHs and PAQs are associated with combustion in both petrol and diesel engines, as well as with non-exhaust traffic sources such as brake linings, tires and road construction materials [19, 32, 33]. They have been detected in atmospheric particles, road dust, and runoff from streams in traffic dense areas [34–37]. These compounds are commonly released in particulate form and have shown strong correlations with rush hour traffic and traffic-related gases e.g., CO and NOX [32, 38]. Studies have also shown a reduction in PAH and PAQ concentrations over time after the introduction of light diesel fuels for buses and the exchange of older petrol-driven passenger cars with catalyst-equipped new ones [39, 40].
- BTs have found extensive use as additives in vehicle antifreeze and corrosion inhibitors, and have been detected in road runoff-impacted stormwater and receiving waters [24, 41, 42]. BTs have been detected in road dust and strong correlations were observed between BT concentrations and road density [24, 41]. 1H–BT, a member of this group, has also been suggested as a suitable marker of metal corrosion from vehicles due to its strong positive correlations with vehicle-related metals—Al, Cu, Fe and Zn—in road dust samples [41].
- We recently implicated the use of BT-UVs in vehicle automotive clear coats and the erosion of those clear coats as well as the potential leaching of BT-UVs from them as a plausible traffic-related source [23]. BT-UVs have been detected in road dust samples from Canada [22] and Japan [43], and Nakata et al [43] further found significant correlations between BT-UV concentrations and road density, suggesting that automobiles were the major source of BT-UVs in road dust.
- OPEs also have traffic-associated sources, such as emissions from vehicle ventilation systems (from their use in automotive furniture) or leakage of motor oils and lubricants [44], and have also been detected in road dust from high traffic areas [45]. While this may explain the similar CQ patterns also observed for OPEs and the other compound groups in figure 1, watershed ratios in figure 2 and regressions in figure 3 suggest that OPEs are more likely to be from both traffic and non-traffic related sources. Based on the lack of significant correlations between OPEs in street dust and factors like population density and vehicle ownership, Pang et al [46] postulates that OPEs in street dust may originate from a combination of various sources including road traffic and atmospheric deposition.
The ready availability of those substances for delivery during storm events, indicated by the clockwise hysteresis in the CQ plots, could be explained by their accumulation on roadways, parking lots, and other impervious surfaces in the dry period antecedent to the precipitation event. The data do suggest a relationship between contaminant delivery to the streams and the antecedent dryness and the imperviousness of the ground. The amount of rainfall in the Mimico watershed in the 2 weeks preceding the rain event in 2018 was about 18 and five times the amount in 2014 and 2015 respectively (table 1). Higher contaminant loads and variable delivery patterns e.g. the appearance of an intense first flush, have been associated with longer antecedent dry periods [20, 21, 47]. The longer antecedent dry periods in 2014 and 2015 likely allowed for the accumulation of contaminated particles, making these contaminants readily available for wash-off. This also explains the discrepant CQ patterns observed at MOL in 2014 and 2015. Also, notwithstanding the lower rainfall amount, the runoff ratio, which assesses the proportion of streamflow comprised of surface runoff, was highest in 2014 (table 1)—a likely result of the frozen topsoil observed during this time [21], which led to enhanced contaminant delivery to the creek.
Some urban water contamination associated with vehicular traffic is well known and well-studied. This includes chemicals in vehicle exhaust, including PAHs, PAQs, volatile organic compounds, heavy metals, NOx , SOx , CO, CO2, hydrocarbons and platinum group elements [48–51], and the use of road salts (including anti-clumping agents such as ferrocyanide, chromate or phosphate) and other deicers used to promote safe driving during the winter season [52, 53]. More recently, attention has been paid to non-exhaust traffic-related emissions from TWPs, brake wear particles (BWPs), road wear particles, and their associated chemicals and by-products, including heavy metals, vulcanization agents, antioxidants, plasticizers, softeners, binders, fillers and other additives [51, 54–56]. These compounds have also been shown to be efficiently transported to nearby streams during runoff events [15, 17, 57, 58]. Although these studies have identified traffic and roads as important emitters of organic contaminants [19, 52, 55, 59], our work was focused on substance groups (BT, BT-UVs, OPEs) for which road traffic has not previously been implicated as a major source. Except for the obvious PAHs, none of the compound groups we studied had been explicitly associated with traffic and roads. As varied and large as the group of organic contaminants was in our studies on urban runoff in Toronto, they likely only comprise a small portion of the organic compounds potentially associated with road traffic and the flushing of road surfaces. Compounds associated with road traffic that could affect urban waters include:
- Tire wear chemicals: Organic chemicals used as additives in tire production have been observed in urban stormwater and receiving waters and have been shown to cause adverse effects on aquatic species. Peter et al found similarities between TWP leachate and road runoff that implicates TWPs as the main source of contaminants in urban runoff responsible for observed lethality in adult coho samples [59]. More recent studies have identified N-(1,3-dimethylbutyl)-N'-phenyl-p-phenylenediamine, a tire rubber antioxidant, 1,3-diphenyl guanidine and hexamethoxymethylmelamine—additives used in tire production—as ubiquitous contaminants in urban receiving waters which also induce coho salmon mortality [15, 56]. The number of potential tire-borne water contaminants is likely much larger [60]. Other contaminants including heavy metals, alkylphenols, phthalates, PAHs, microplastics, and benzothiazoles have also been identified as plausible emissions from tire wear in trafficked environments [19, 57, 61].
- Brake wear chemicals: Brake linings of vehicles contain a mixture of fillers, binders, fibers, lubricants, and abrasives [55], all of which contain organic and inorganic chemical components that can be released during wear. Alves et al recently found more than 150 organic compounds, including aliphatics, PAHs, alcohols, acids, glycol/glycerol derivatives, plasticizers, sugars, sterols, and various phenolic constituents in particles derived from brake wear [62]. Other studies have further found BTEX, PAQs and heavy metals in tests of BWPs [19, 55], all of which are contaminants that could potentially end up in receiving waters.
- Poly- and per-fluoroalkyl substances: The presence of poly- and per-fluoroalkyl substances in street dust in heavily trafficked areas has been repeatedly established, implicating traffic-related materials such as automotive grease, car seats, steering systems, and brakes as the source of these contaminants which eventually end up in surface water [5, 63–66].
- Chemicals from road construction: In addition to heavy metal releases from TWPs and BWPs, road construction materials, such as the yellow paint used for marking the division of lanes on roads can be a source of heavy metals in road dust, and thus in urban runoff. In particular, Pb and Cr have been identified as heavy metals in road dust sourced from the yellow lane divider paint [67, 68]. Other contaminants like phthalates, alkylphenols and alkylphenol ethoxylates, bisphenol A, PAHs, antioxidants, and microplastics have been associated with road construction materials including bitumen, asphalt, concrete and road paint [19, 69]. Abrasion of roads by repeated contact with vehicle tires can generate road surface particles over time which become incorporated into road dust.
- Emissions from vehicle components: Other vehicle components such as body paint, coatings, plastic components, furniture and engines also undergo wear and tear during their lifetime and can deposit pollutants such as metals, phthalates, PAHs, BT-UVs and flame retardants on road surfaces [19, 23, 44, 52].
- Vehicle fluids and car care products: Lubricants, grease, antifreeze, motor oil, windshield deicers, and other fluids used in vehicles are subject to leakages, especially due to poor vehicle maintenance, and can be carried by stormwater into urban waters. These fluids can contain volatile organic compounds (e.g., ethanol, methanol, 2-methoxy ethanol, polyethylene glycols, octylphenol ethoxylates and polypropylene glycols [55, 59]), heavy metals [70], and flame retardants [44].
This long list highlights that the problem of urban water contamination with organic compounds arising from road traffic is more complex and pervasive than generally thought.
The fleet of vehicles on most roadways globally will undergo a dramatic shift over the next two decades, largely propelled by the switch from internal combustion engine vehicles (ICEVs) to electric vehicles (EVs). This electrification will undoubtedly result in the decline in roadside emissions of chemicals associated with ICEVs, including exhaust-related (combustion by-products such as the PAHs) and non-exhaust-related (e.g., gasoline components, other chemicals associated with combustion engines, e.g. motor oils and their additives). Because the energy recuperation of EVs reduces the use of brakes, one might even expect a reduction in brake pad wear [54, 71]. However, other non-exhaust vehicle emissions e.g., tire wear, road surface wear and particle resuspension (which frequently exceed exhaust emissions) are projected to not change by much or to even increase. The increased weight of EVs due to the batteries has been linked to similar and higher particulate matter (PM2.5 and PM10) emissions [55, 72]. Non-exhaust emissions of volatile organic contaminants from solvents used in ancillary systems and for car care e.g., screen wash and deicing fluids, may persist even with the introduction of EVs [55], or possibly change as a result of climate change (less use of deicers).
As we show in this study, traffic may be primarily responsible for the delivery of multiple organic contaminant groups to urban streams via non-exhaust sources. This suggests that any improvement in urban water quality potentially anticipated as a co-benefit of vehicle fleet electrification may not necessarily materialize. Whereas many studies have shown the impact, or lack thereof, of vehicle fleet electrification on urban air quality [72–74], studies are scarce on the impact on urban water quality. However, mitigation measures such as the use of on-vehicle brake wear capture, development of wear-resistant tires, road surface cleaning, and the application of dust suppressants to road surfaces, which have been proposed for improving urban air quality [55], can also be applied to improve urban water quality, especially since many stormwater contaminants are mostly particle-bound. Hixon et al [75] indeed show that street sweeping is potentially significant in reducing downstream pollutant loads in urban watersheds. Other control measures, such as the use of stormwater retention ponds, detention basins, and swales can also mitigate the transport of chemicals from roads to receiving waters [51]. Finally, raising awareness, and implementing regulations and/or incentives for promptly fixing leaky vehicles can be a useful avenue to reduce the amount of vehicle fluids making their way into rivers via urban stormwater [76].
5. Conclusion
By combining years of data collected on the transport of various organic contaminants to urban streams receiving no direct wastewater or sewage input, we found that multiple compound groups have similar transport pathways, pointing to similar sources and delivery mechanisms. Traffic and vehicle-related sources, to which PAHs, PAQs, BT-UVs, and BTs are all associated are shown to be a plausible common source of these contaminants to urban surfaces, and to receiving urban streams following runoff. OPEs, which are also traffic-related, show a greater diversity in sources, while the behavior of herbicides clearly reflects their predominant use in agricultural areas. The importance of traffic and road surfaces as a source of a myriad of organic contaminants to urban creeks and rivers is becoming increasingly recognized and is deserving the full attention of those tasked with the health of urban waters.
Acknowledgments
We acknowledge funding from a Discovery Grant from the Natural Sciences and Engineering Research Council of Canada (NSERC).
Data availability statements
The data that support the findings of this study are openly available. They can be found in [20–26]. The DOI for these datasources is given in the reference list.
Conflict of interest
The authors declare no conflict of interest.