Abstract
The availability of water is a growing concern for flooded rice production. As such, several water-saving irrigation practices have been developed to reduce water requirements. Alternate wetting and drying and mid-season drainage have been shown to potentially reduce water requirements while maintaining rice yields when compared to continuous flooding. With the removal of permanently anaerobic conditions during the growing season, water-saving irrigation can also reduce CO2 equivalent (CO2eq) emissions, helping reduce the impact of greenhouse gas (GHG) emissions. However, the long-term impact of water-saving irrigation on soil organic carbon (SOC)—used here as an indicator of soil health and fertility—has not been explored. We therefore conducted a meta-analysis to assess the effects of common water-saving irrigation practices (alternate wetting and drying and mid-season drainage) on (i) SOC, and (ii) GHG emissions. Despite an extensive literature search, only 12 studies were found containing data to constrain the soil C balance in both continuous flooding and water-saving irrigation plots, highlighting the still limited understanding of long-term impacts of water-saving irrigation on soil health and GHG emissions. Water-saving irrigation was found to reduce emissions of CH4 by 52.3% and increased those of CO2 by 44.8%. CO2eq emissions were thereby reduced by 18.6% but the soil-to-atmosphere carbon (C) flux increased by 25% when compared to continuous flooding. Water-saving irrigation was also found to have a negative effect on both SOC—reducing concentrations by 5.2%—and soil organic nitrogen—potentially depleting stocks by more than 100 kg N/ha per year. While negative effects of water-saving irrigation on rice yield may not be visible in short-term experiments, care should be taken when assessing the long-term sustainability of these irrigation practices because they can decrease soil fertility. Strategies need to be developed for assessing the more long-term effects of these irrigation practices by considering trade-offs between water savings and other ecosystem services.
Export citation and abstract BibTeX RIS

Original content from this work may be used under the terms of the Creative Commons Attribution 3.0 licence. Any further distribution of this work must maintain attribution to the author(s) and the title of the work, journal citation and DOI.
1. Introduction
Food security is one of the most pressing challenges facing society. With a growing global population, expected to reach 9 billion people by 2050, 50% more food than currently produced may be required to meet future demands (Maraseni et al 2018). The challenge of meeting future production requirements is complicated by increasing demands on limited natural resources that are also essential within multiple sectors (i.e. energy production, industrial and domestic uses), and for ecosystem functions. Of course, this challenge also needs to be considered in the context of climate change, the effects of which are beginning to become a reality for the agricultural sector (Deutsch et al 2018).
The production of cereal crops faces much of the burden of meeting future food provision, with rice currently representing 19% of human calorific intake (FAOSTAT 2018) and being a staple food for 3.5 billion people. Water use is one of the major challenges for sustainable production of rice. The semi-aquatic nature of the plant's ancestors results in the crop having limited tolerance to water stress (Kögel-Knabner et al 2010). Rice is thus predominantly grown in irrigated lowland conditions, which account for 75% of global rice production (Bouman et al 2007). In these systems, the crop is grown in bunded fields that are flooded for the majority of the growing season (i.e. continuous flooding). Due to the flooded nature of production and the importance of rice as staple food, rice agriculture accounts for up to 30% of global freshwater withdrawals (Bouman et al 2007).
Meeting the large water requirements of rice has historically not been a concern for farmers due to the location of production being in water-abundant areas. In the past, seasonal rains and predicable floods have allowed for the natural flooding of fields or for irrigation water to be withdrawn from rivers or groundwater sources with little concern for excessive water depletion or upstream/downstream abstraction issues. Yet, as agricultural production and other sectors demands increase, water scarcity and reduced water quality are becoming sources of concern in many regions (Bouwer 2000, Bouman and Tuong 2001, Liu et al 2017).
Solutions for managing water scarcity in rice production need to maintain yields while reducing water consumption. Alternate wetting and drying and mid-season drainage, among other water saving irrigation strategies, have been posed as potential strategies that could achieve these aims. Numerous short-term experiments have investigated the viability of these technologies, with mixed results. A meta-analysis investigating the impact of alternate wetting and drying on yield showed that yields could be maintained, but this depended on soil characteristics and the severity of the soil drying that was implemented (Carrijo et al 2017).
Irrespective of changes in water management, yield stagnation or decline is a growing concern for major crops (Ray et al 2012), including rice (Dobermann et al 2000, Ladha et al 2003, Van Nguyen and Ferrero 2006). With the intensification of rice production, and the introduction of multiple annual harvests or rice-upland crop production, concerns have been raised regarding the sustainability of high yields. Yield declines might have been partly caused by climate change, which may have off-set yield improvements stemming from, among others, improvements in technology and carbon dioxide (CO2) fertilization (Lobell et al 2011). However, declining soil fertility has also been suggested as a likely cause for yield stagnation (Dobermann et al 2000).
In a changing climate, agriculture is simultaneously a sector that contributes to global warming (Robertson et al 2000) while also being posited as a potential solution to mitigate increasing atmospheric CO2 (Zomer et al 2017). Rice agriculture has important climate warming implications due to the emission of non-CO2 greenhouse gasses (GHG). While climate warming is commonly discussed in terms of rising CO2 concentrations, methane (CH4) and nitrous oxide (N2O) are also of concern due to their higher warming potential. In paddy rice systems, the predominantly anaerobic conditions during the growing season lead to rice production being a significant emitter of CH4, accounting for approximately 11% of anthropogenic CH4 emissions (Heinze et al 2013). As such, water-saving irrigation could contribute to CH4 emission reduction (Wassmann et al 1993), although this benefit may be partially off-set by increased N2O emissions (Kritee et al 2018). Water-saving irrigation may also increase CO2 emissions from rice cultivation. As soils become non-saturated, aerobic conditions prevail, and heterotrophic respiration results in increased mineralization of SOC (Moyano et al 2013). As such, changes in water management in rice agriculture have consequences also for the SOC balance and more broadly for soil fertility.
Considering the relatively slow, often decadal pace, at which soil properties change, the lack of suitable long-term data may lead to misjudgements of the consequences of land management (Magnuson 1990). Despite the need to understand the effects of water-saving irrigation on global warming and SOC stocks, these aspects have not been sufficiently studied (Haque et al 2017). Reductions in CO2 equivalent emissions (CO2eq), and changes to the emission of individual GHGs, are the result of changing SOC dynamics, with possible consequences for C storage in paddy soils. Paddy soils are often considered a C sink (Liping and Erda 2001), with SOC concentrations and stocks larger than local background levels (Pan et al 2010, Wu 2011, Chiti et al 2012, Lehndorff et al 2016). By improving soil aeration, water-saving irrigation may lead to a loss of SOC, potentially turning paddy rice soils from carbon sinks to sources (figure 1).
Figure 1. Conceptual framing of changes in SOC as a result of water-saving irrigation in: (a) continual flood irrigation; and, (b) alternate wetting and drying and mid-season drainage. Decreased water use increases aerobic soil conditions, reducing CH4 emissions while simultaneously increasing those of CO2, resulting in either decreased accumulation rates or loss of SOC.
Download figure:
Standard image High-resolution imageThe long-term sustainability of rice yields is dependent on soil fertility, which in turn correlates with SOC (Lal 2005). Therefore, the loss of SOC caused by changes in water management may lead to yield reductions (Grace et al 2003). Quantifying SOC losses is thus important to predict impacts of water-saving irrigation on yield and yield growth, as well as GHG emissions and global warming potentials. Only in this way is it possible to account for trade-offs that are implicit in the choice of the water management strategy. At present, research has commonly focused on changes in GHG emissions—in particular CH4 and N2O. Little attention has been devoted to understand the effect of water-saving irrigation on the soil C balance. Previous studies have principally focused on the effect of water-saving irrigation on yield, but over time-scales which are not sufficiently long enough for potential changes in soil properties to have an effect. The average experiment timescale of studies analyzed in a recent meta-analysis (Carrijo et al 2017) was between one and two years. This period of time is unlikely to capture the dynamics of physical, biological and chemical processes which will determine SOC and yields over decades. In this regard, concluding that improved water management can be achieved whilst sustaining yields holds only for the short-term.
To fill this gap, this study aims to understand the impacts of shifting from continuous flooding production to mid-season drainage and alternate wetting and drying on the soil C balance, through assessment of changes in the emission of CH4 and CO2, which may signal potential changes in soil carbon stocks, and through direct measurement of changes in SOC. It also aims to understand if changes in GHG emissions from water-saving irrigation have net positive effects on the global warming potential of rice production. Through a meta-analysis, we ask:
- 1.Do alternate wetting and drying and mid-season drainage lead to reductions in SOC compared to continual flooded irrigation?
- 2.Are the climate warming benefits of CH4 reductions off-set by increased emissions of N2O and CO2?
2. Methods
2.1. Literature search
To assess the impact of water-saving irrigation practices (alternate wetting and drying and mid-season drainage) on SOC, a literature search was conducted to gather articles reporting changes in SOC from split plot experiments where either of the two water-saving irrigation practices were compared against continuous flooding irrigation. The literature search was conducted within Web of Science, on 26th November 2018. The following string was used: ((rice OR paddy OR 'oryza sativa' OR 'oryza s*') AND (SOC OR 'soil carbon' OR 'soil organic carbon' OR 'soil c' OR 'organic carbon' OR 'carbon sequestrat*' OR 'C sequestrat*' OR 'soil organic C' OR 'carbon pool' OR 'carbon stock' OR 'carbon storage' OR 'soil organic matter') AND (water OR irrigat* OR 'water management' OR flooded OR anaerobic OR aerobic OR 'alternate wetting and drying' OR AWD OR 'intermittent wetting and drying' OR MSD OR 'midseason drainage' OR 'mid-season drainage')).
The search returned 1680 research articles. These articles were then sifted through a systematic title and abstract screening to exclude articles which were: (1) not related to irrigation of rice that could be equated to either alternate wetting and drying or mid-season drainage; (2) were not field based. Articles were kept in instances where it was unclear whether they met the two exclusion factors detailed above. The title and abstract screening resulted in the identification of 56 articles. These 56 articles were then assessed through reading of their content, including supplementary material, and were retained for analysis if they reported SOC concentrations for each water treatment at the end of at least one season, resulting in seven articles being carried forward into the analysis.
As changes in SOC are affected by emissions of both CH4 and CO2 (figure 1), a second search was conducted 5th December 2018 to identify articles reporting emissions of both gases. The search string for this search was: ((rice OR paddy OR 'oryza sativa' OR 'oryza s*') AND ('carbon dioxide' OR methane OR CO2 OR CH4 OR minerali* OR respiration) AND (water OR irrigat* OR 'water management' OR flooded OR anaerobic OR aerobic OR 'alternate wetting and drying' OR AWD OR 'intermittent wetting and drying' OR MSD OR 'midseason drainage' OR 'mid-season drainage')), returning 4377 results. As with the first search, articles were then sifted to exclude those that were not related to alternate wetting and drying or mid-season drainage irrigation of rice, and were not field based, identifying 59 articles. These 59 articles were then assessed and were included in the analysis if they reported cumulative CH4 and CO2 emissions for each water treatment at the end of at least one season. This assessment resulted in seven articles being retained for analysis.
In both searches, only articles where all other aspects of the treatments (chemical fertilization, organic amendments, residue retention, planting method, tillage, initial soil properties, etc) are the same between control and treatment, and only water management is adjusted were kept.
As we also sought to assess changes in CO2eq emissions, which are the product of changes to CH4, CO2, and N2O, from the introduction of water-saving irrigation, the second literature search was also used to identify articles reporting cumulative N2O emissions. Although the search string did not explicitly mention key words for identifying articles reporting N2O emissions, it was assumed that relevant articles would be captured by the search string (i.e. articles reporting N2O emissions alongside CH4 and CO2 would appear in the search results).
For each identified study, primary data were collected, including initial and final SOC concentrations, BD, cumulative growing season GHG emissions, study period, experimental replication, grain yield and water use. Secondary data of potentially regulating variables were also recorded, including soil texture, pH, total nitrogen, total phosphorus, chemical and organic fertilizer additions, tillage practices, average and annual mean temperature and precipitation, and geographic coordinates. While the intention was to assess changes in SOC stocks and GHG emissions in relation to secondary variables, the limited number of articles found and the inconsistent reporting of the secondary variables in those articles prevented us from assessing their regulating effect on stocks or emissions.
2.2. Data collection and preparation
In studies that ran over multiple years or seasons only SOC concentrations after the final harvest within the study were recorded. In these studies, SOC concentrations were collected for the first 20 cm of soil or to the deepest depth that could be obtained if sampling within an individual study was not conducted down to 20 cm.
The effect of alternate wetting and drying has been tested at many different drying thresholds. Here, if more than two drying thresholds were used in any single study, only results from the two least extreme drying levels were used. Where GHG monitoring was conducted over multiple seasons, the average gas flux for the monitoring period was used to avoid over-weighting results from studies which ran over more than one season compared to single season studies. As some studies tested the effect of water-saving irrigation in relation to different quantities of organic additions or chemical fertilizers, if these additions were tested in both continuous flooding and alternate wetting and drying or mid-season drainage, each level of addition was treated as a pair.
To evaluate the compound effect of the GHG emitted from flooded rice fields, i.e. CO2, CH4 and N2O, we calculated CO2eq emissions as the sum of emissions from the three individual gasses, multiplied by their respective IPCC 100-year emission factors (Myhre et al 2013):

The direction of the change in C emissions is relevant to our understanding of changes in SOC. Changes in SOC stocks result from the difference between input of organic C and output of CO2, CH4, and dissolved organic C (not quantified here due to lack of data). To estimate C emissions on a mass basis (consistent with measured changes in SOC), CO2 and CH4 fluxes were converted to C-mass fluxes considering the molar ratios of CO2 and CH4.
Differences in soil organic nitrogen (SON) stocks between treatment pairs were estimated from SOC concentrations, bulk density (BD), and C:N ratios provided in the studies for each treatment. SOC stocks were estimated using reported SOC concentrations and estimated BD. When BD was not reported, the average value from all studies (1.3 g cm−3) was used. Despite some variability in the organic matter C:N ratio across studies (6.6 to 12.4 g C/g N), changes across treatments were small and inconsistent. Therefore, in the three studies lacking information on SON, we used the average C:N ratio from all reporting studies.
The effect of 30 year (1987–2016) mean annual precipitation (P), potential evapotranspiration (PET), temperature (T) on SOC changes was also explored. Mean annual climatic variables were obtained using the CRU TS 4.01 P, T, and PET gridded data products (Harris et al 2014), and reported site locations.
2.3. Statistical analyses
For each study, response ratios, i.e. the ratio between treatment (alternate wetting and drying or mid-season drainage) and control (continuous flooding), were calculated for SOC concentrations, cumulative seasonal GHG emissions (CH4, CO2, N2O), CO2 equivalent (CO2eq), and total C emissions (the sum of C in CH4 and CO2 fluxes). The effect size of the water management treatments was then determined through the natural logarithm of the response ratio, R:
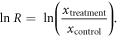
The arithmetic mean and 95% confidence interval were then calculated, where the confidence interval is based on Student's t-distribution due to the small sample size. Results were then back-transformed, taking the exponent of the arithmetic mean and confidence interval, giving the geometric mean and its corresponding confidence interval. This allowed for results to be expressed as percent change, here termed effect size. All studies collected had between three and four replicates, but neither standard deviation nor standard error were consistently reported. In other meta-analyses where standard deviation, standard error or the number of replications were not consistently reported, equal weights were given to each sample set (Johnson and Curtis 2001, Gopalakrishnan et al 2014, Qin et al 2015, Han et al 2016). The same approach was followed here.
3. Results
The distribution of study locations can be seen in figure 2 (see also supplemental table 1 available online at stacks.iop.org/ERL/14/074011/mmedia). Numerous studies have reported the effect of alternate wetting and drying or mid-season drainage on emissions of CH4 and N2O in rice production (Yang et al 2016). However, only seven studies (Haque et al 2016, Haque et al 2017, Kumar et al 2016, Tang et al 2018, Wang et al 2017, Xu et al 2016, Xu et al 2017), giving 16 paired observations, also reported CO2 emission alongside CH4 in paired fields irrigated by continuous flooding and alternate wetting and drying or mid-season drainage. Two studies (Haque et al 2016, 2017) reported effects of irrigation on both GHG and SOC. In total, seven studies assessed changes in SOC concentrations (Haque et al 2016, Haque et al 2017, Tirol-Padre et al 2018, Wu et al 2018, Xu et al 2017, Yang et al 2005, Yang et al 2018), covering locations in six countries, resulting in 17 paired observations. All GHG studies were conducted over either one or two growing seasons, but those investigating changes in SOC varied greatly in length, with six studies covering between 1 and 4 years and one 17 years.
Figure 2. Locations of study sites identified within the literature search where either soil organic carbon (SOC), greenhouse gas (GHG) emissions, or both were reported.
Download figure:
Standard image High-resolution imageFigure 3(a) shows seasonal emissions of CH4 were reduced when adopting water-saving irrigation practices, while both N2O and CO2 emissions increased. Despite the fact that increased CO2 and N2O emissions offset some of the benefits gained from reduced CH4 emissions under water-saving irrigation, CO2eq emissions were on average decreased under water saving irrigation. The mean percentage changes of emissions under water-saving irrigation compared with continuous flooding (and confidence intervals) for CO2eq, CH4, CO2, N2O were −18.6% (−24.9 to −11.9), −52.3% (−63.2% to +38.1%), +44.8% (+29.2% to +62.2%), and +37.0% (+26.0% to +48.8%), respectively (figure 3(a)).
Figure 3. Combined effect size of alternate wetting and drying and mid-season drainage with respect to continuous flooding on (a) greenhouse gas emissions, and combined carbon dioxide equivalent (CO2eq); and (b) soil organic carbon (SOC) concentrations and total carbon emissions (Cemit). Markers represent the geometric mean (horizontal dash), and the 95% confidence interval (vertical line).
Download figure:
Standard image High-resolution imageFigure 3(b) shows the effect of water-saving irrigation on SOC concentrations and C emissions. While CO2eq emissions were governed by CH4 and N2O emissions, carbon emissions on a mass basis were controlled primarily by increased CO2 production under water saving irrigation. Higher C emissions may lead to SOC loss when increased emissions are not balanced with increased C input, which was the case in this study, i.e. a consistently negative effect of alternate wetting and drying and mid-season drainage on SOC. The mean percentage change (and confidence interval) of C emissions and SOC concentrations were +24.6% (+14.5% to +35.5%), and −5.2% (−8.9% to −1.3%), respectively (figure 3(b)).
The range of estimated changes in SON was large and varied from −362 to +743 kg N/ha per year, where the negative values represent N gains. Losses of organic N associated to soil organic matter across the analyzed studies amounted to 138 kg N/ha per year on average, approximately equivalent to the typical fertilizer application rate in the seven studies reporting changes in SOC concentrations. The results suggest a trend in the direction we expected, though not significant (p = 0.08).
We performed linear regression analysis to explore the potential effect of the climatic variables PET, T, P on effect size (supplement figure 1). Only 10% and 15% of the variation could be explained by the regressions lines, which had insignificant slopes (p > 0.05) for T and PET. P was found to have a significant positive effect on SOC response ratios (p < 0.05), with R2 = 0.5.
4. Discussion
4.1. Effects of water management on GHG emissions
Only seven studies were found which reported CO2 alongside CH4 emissions in paired rice fields under different water managements. All studies demonstrated a consistent trend of increased CO2 emissions coupled with decreased CH4 emissions. Despite the limited number of data points, the change in CH4 emissions (−52.3%) is consistent with those reported elsewhere (e.g. approximately −50%, in the meta-analysis by Sanchis et al (2012), and −53% by Jiang et al (2019)). This consistency suggests that, while little work has been done on CO2 emission responses to water-saving irrigation (Yang et al 2017), the mean 44.8% increase in CO2 emissions found here may be a reasonable estimate also for other sites. However, the mean increase in N2O emissions in this study is lower than the mean increase of 102%, but within the confidence interval, found by Jiang et al (2019). The difference across studies may be due to the highly episodic nature of N2O emissions not being captured by the relatively coarse gas measurement designs of the seven studies used within this meta-analysis. Only two of the seven studies conducted gas samping on a daily basis, with the others being between twice per week and every 10 d. Within the five studies that sampled less than once per day, only two intensified the sampling during fertilization or during drying events. Therefore, these studies may have not captured periods of high N2O emissions.
Water-saving irrigation reduces emissions of CH4, having a benifical effect on total GHG emissions. However, this benificial effect is partially negated through increased emissions of CO2 and N2O. Shifting from continuous flooding to alternate wetting and drying or mid-season drainage reduces water demand and global warming potential. While both N2O and CO2 emissions increase and off-set some of the benefits of reduced CH4 production, water-saving irrigation reduced CO2eq emissions (figure 3(a)). Nitrous oxide has the greatest warming potential of the three reported gases, but actual emissions was very low and not sufficient to negate the benefit of CH4 reductions. Conversely, while the increase in actual emissions of CO2 was much greater than the reduction in CH4, the greater warming potential of CH4 resulted in a more marked effect on the CO2eq emissions than CO2. Therefore, from a greenhouse warming perspective, switching from continuous flooding to alternate wetting and drying or mid-season drainage potentially reduces the impact of rice production on climate change.
Recent work has criticized the use of CO2eq in policy setting (Allen et al 2018). Short-lived and long-lived GHGs affect the trajectory to, and recession from, peak warming. The replacement of CH4 emissions with those of CO2 and N2O does not necessarily provide a positive outcome in regards to mitigating warming. Therefore, while CH4 reductions have often been highlighted as a benefit of water saving irrigation, this conclusion can only be made with full consideration of the conseqences on present and future emissions. As such, care must be taken in stating the positive benefits of CH4 reductions in regard to climate change mitigation.
4.2. Effects of water management on SOC
Maintaining yields and reducing the global warming potential, while simultaneously reducing water inputs (up to about 30%; Carrijo et al 2017) are all positive consequences of improved water management. The increased emission of CO2 seen in figure 3(a) was not large enough to negate the positive effect that water-saving irrigation has on reducing CO2eq emissions, but it signals a departure between the SOC concentrations in continuous flooding and alternate wetting and drying or mid-season drainage fields. As the gaseous C loss is mainly due to CO2, the soil-to-air C flux under water-saving irrigation was significantly increased (figure 3(b)), suggesting a reduction in SOC concentration compared to continuous flooding. This is further confirmed by the 5.2% decrease in SOC concentration shown in figure 3(b).
Due to limited reporting of changes in BD in the selected studies, it was not possible to calculate changes in SOC stocks. Yet, the observed increases in CO2 emissions suggest that, regardless of changes in BD, water-saving irrigation can result in either an absolute reduction in SOC stocks (rice fields becoming a C source) or a reduction in C sequestration rates compared to flood irrigation (becoming a weaker C sink). The consequence of this depends on the severity of the drying, the state of the SOC stock, additions of organic and chemical fertilisers, and the C storage potential. As SOC concentrations follow saturation curves, which in turn are dependent on the above stated factors, the carbon loss or gain rate slows as the system approaches a new equilibrium. In all the reviewed studies, alternative irrigation approaches have been adopted for between 1 and 17 years. Despite the short duration of most studies, the mean reduction in SOC under alternate wetting and drying or mid-season drainage is statistically significant, suggesting that even larger SOC losses may occur in the long-term. However, the overall long-term consequence is site specific, making it difficult to anticipate whether long-term trends in SOC will begin to have an effect on yields.
The significant effect of precipitation on effect size may be a consequence of the native saturation capacity of the soils at the study sites. SOC has been shown to be positively and linearly correlation with precipitation (Chang et al 2014). Flooded rice production may have caused larger accumulation of SOC in relatively drier sites, where the background SOC was lower. Thus, the removal of flooding and the establishment of water-saving irrigation practices may have a more marked effect on SOC loss in these locations, where SOC in paddy fields is disproportionally higher than in non-irrigated land.
4.3. Effects of water management on organic nitrogen
Flooded rice systems enable the accumulation of SON (Ladha et al 2011), which may be lost through leaching in water-saving irrigation (Tan et al 2013). The approximate calculation of organic N losses (138 kg N/ha per year on average when assuming no increase in BD) highlights the risk of losing large amounts of nutrients that were chemically bound in organic matter when continuous flooding is substituted by water-saving irrigation. In low fertilization systems that rely on N supply from soil organic matter, a part of the lost nutrient stocks could have supported crop growth. Further, where the loss will result in leaching, increased N loads to water bodies may cause eutrophication. However, leaching may also be reduced due to decreased percolation in water saving irrigation compared to continuous flooding (Peng et al 2011).
4.4. Implications for agricultural management
While potentially being beneficial for reducing the global warming potential of rice production, the impacts of improved water management may extend beyond the other commonly reported benefit of water saving. The introduction of aerobic conditions during the growing period alters soil processes and chemistry. The consequences of these changes need to be fully understood to determine the sustainability of improved water management. Here, we highlight the consequences of water-saving irrigation on SOC and the impact this may have on long-term production. These long-term consequences may not be immediately experienced, but attention should be paid to their potential impact.
While few studies have assessed changes in SOC as a result of alternate wetting and drying or mid-season drainage, much attention has been placed on the capacity of these water management strategies to reduce water consumption without sacrificing yields. The meta-analysis by Carrijo et al (2017) found that mild-alternate wetting and drying increased water productivity (grain yield per unit water) by 24% without significant decrease in yields. The same study also identified that the effect of water management is dependent on soil characteristics and the severity of soil drying. Notably, SOC concentration played a role in limiting yield reductions, where the impact of alternate wetting and drying on yield was lower when SOC concentrations were above 1%. Elsewhere, SOC has also been positively correlated to yield (Pan et al 2009), with paddy rice systems often promoting SOC sequestration (Pan et al 2010). Conversely, cessation of paddy rice production has also been linked to a rapid loss of SOC (Desjardins et al 2006, Chen et al 2017). Our results and this evidence point consistently to water-saving irrigation causing reduction in plant-available nutrients, which may, in the long-term, contribute to yield declines.
To counteract SOC and nutrient losses, management strategies could be adjusted to increase the amount of biomass being returned to the soil. This goal can be achieved via additions of crop residues (Liu et al 2014), organic amendments (Kätterer et al 2011), chemical fertilizers (Geisseler et al 2017, Poeplau et al 2018), and changes to tillage practices (Zhang et al 2014). However, without first recognizing and quantifying the risk of SOC loss, appropriate countermeasures cannot be identified.
Consequences of water-saving irrigation extend beyond soil properties. There are clear short-term benefits to individual farmers from improved water productivity. There are also site-specific benefits, such as reduced lodging (Yamaguchi et al 2017), and community benefits through more equitable water allocation between farms (Lampayan et al 2015). Conversely, there are potentially long-term effects to the ability of soils to maintain yields, due to the slow response of these soils to changes in land management. Moreover, flooded rice fields are multi-functional systems that provide, for example, habitats for fish species—a saleable protein source and pest control (Berg et al 2017). These multi-functional benefits may be lost through increased management of water resources resulting from water-saving irrigation, but these long-term effects—as those for soil fertility—are still poorly characterized.
5. Conclusions
Our results point to a previously under-recognized trade-off between water and SOC management, that reduced water consumption when applying water saving irrigation may have negative consequences on soil organic matter and fertility. Despite the limited number of studies identified in the literature, water-saving irrigation was found to reduce concentrations of SOC when compared to flood irrigation. Water-saving irrigation was also found to decrease CH4 emissions in a manner consistent with reductions reported elsewhere, and these reductions were coupled with increased CO2 and N2O emissions. Despite the overall decline in GHG emissions, the increased production of CO2 resulted in increased emission of C from water-saving irrigation soils compared to those under flood irrigation. The associated change in SOC concentrations may reduce soil nutrient availability, which could affect the ability of soils to maintain rice yield in the long term. Present studies on the effect of water-saving irrigation on yields are generally too short (1–2 years) to assess the effect that changes in soil properties may have. In rice fields where alternative water managements allow for beneficial reductions in water consumption, these benefits may be lowered as a result of organic matter loss, having negative consequences on yield sustainability. Given the short time scale of the available studies, from which we have drawn our conclusions, we can only surmise that there is a risk for a long-term negative yield trade-off. Since extrapolating conclusions from short term soil experiments is challenging (Richter et al 2007), the long-term impact of water-saving irrigation practices in different rice-producing regions should be addressed in future studies.
Acknowledgments
This work was supported by the Swedish Research Council (Vetenskapsrådet), Formas, and Sida through the joint call 'Sustainability and resilience—Tackling climate and environmental changes' (VR 2016-06313). We thank Jan-Olof Persson for his statistical advice, and Md Mozammel Haque for the provision of original data. GV gratefully aknowledges also the support of the Swedish Research Council (Vetenskapsrådet), under grant 2016-04910.