Abstract
In this work, new stable and efficiently bio-active lipid nanocarriers (NLCs) with antioxidant properties have been developed for the transport of active ingredients in food. The novel NLCs loaded with β-sitosterol/β-sitosterol and green tea extract (GTE) and prepared by a combination of natural oils (grape seed oil, fish oil and squalene) and biological lipids with food grade surfactants, were physico-chemically examined by DLS, TEM, electrokinetic potential, DSC and HPLC and found to have main diameters less than 200 nm, a spherical morphology, excellent physical stability, an imperfect crystalline lattice and high entrapment efficiency. The novel loaded-NLCs have demonstrated the potential to develop a high blocking action of chain reactions, trapping up to 92% of the free-oxygen radicals, as compared to the native β-sitosterol (AA%=36.5). Another advantage of this study is associated with the quality of bio-active NLCs based on grape seed oil and squalene to manifest a better sitosterol—sustained release behaviour as compared to their related nanoemulsions. By coupling both in vitro results, i.e. the enhanced antioxidant activity and superior release properties, this study emphasizes the sustainability of novel bio-active nanocarriers to gain specific bio-food features for development of functional foods with a high applicability spectrum.
Export citation and abstract BibTeX RIS
1. Introduction
The potential benefits of nanotechnology have been recognized by many industries and commercial products are already being manufactured. In contrast, the application of nanotechnology within the food sector is rather new, even though it presents the potential to influence many aspects of food systems. Up to the present, there have been a limited number of research studies that have been focused on nano-applications in this field, which could have a great chance to be commercially viable now and in the near future. Most developments in food nanotechnology occurred after 2005, since groups of researchers around the world reported their achievements and discoveries about the potential of nanotechnology in the food industry. There are several subdomains where food nanotechnology has been explored [1, 2], but the part that comes in direct contact with the consumer is the development of effective delivery systems suitable for the transport of active ingredients in food. The application of encapsulation systems in food active ingredient delivery has been discussed and reviewed significantly over the last few years [3]. Among the first nanoencapsulation systems were nanosystems based on polymers [4]. Self-assembling structures of microemulsion type [5] and mesophases of liotrope liquid crystals [6] have already been investigated for their potential in the solubilization of lipophyl nutrient molecules. The current use of the above-mentioned delivery systems in food is determined by their processes of preparation, which may involve solvents and high levels of non-food surfactants [7]. In spite of successful elaboration of these delivery systems for biomedical sectors, they have encountered some difficulties for food applications that require compounds generally recognized as safe (GRAS) [8].
As a result, for applying nanoencapsulation in food, and assuring safety regarding possible risks, the solid lipid nanoparticles (SLNs) and nanostructured lipid carriers (NLCs) initially developed in cosmetic and pharmaceutical industries to deliver the lipophyl compounds [9] are appropriate systems for protection and distribution of food compounds.
The nanoencapsulation of bio-active food compounds is an essential requisite for the production of functional food designed to improve the long-term health and well-being of consumers worldwide [10]. Bio-active food compounds known under the name of 'nutraceuticals', refers to nonessential biomolecules that typically occur in small quantities in food and exhibit the capacity to modulate one or more metabolic processes [11]. Their presence as natural constituents in food supplies health benefits in addition to the basic nutritional value of the food product [12]. The effectiveness of nutraceutical compounds in preventing diseases depends on preserving its bioavailability. Unfortunately, most nutraceuticals are highly lipophyl so present poor absorption and limited bioavailability [13]. More importantly, many of these compounds once extracted from animal or vegetal sources are chemically unstable. This represents a major problem, given that only a small proportion of molecules remains available, due to insufficient gastric resistance time and instability under conditions encountered in food processing or in the gastro-intestinal tract (pH, enzymes, other nutrients) [14].
Owing to the greater surface area of lipid nanoparticles per mass unit, they offer promising means for improving the bioavailability of nutraceuticals, especially for poorly soluble compounds such as functional lipids (e.g. carotenoids, phytosterols, ω-3 and ω-6 fatty acids) and numerous other substances that are widely used as active ingredients in various food products. The features of SLNs and NLCs for delivery of nutraceuticals are mainly related to their absorption promoting properties (e.g. they enhance the absorption of nutraceuticals in the stomach and in the small intestine where the food is absorbed) and improved safety. (SLNs and NLCs are physiologically accepted concerning their excipients status [15].)
The field of lipid nanoparticle delivery systems for nutraceuticals with poor water solubility has been significantly growing over the last six years. The first publication that opened the research in this field was by Müller [16] who described the main features of the NLC and SLN systems for oral distribution of active food nutrients. Other researches have treated similar aspects related to the structural design principles of bio-active component delivery in functional foods [17] and the necessity to use lipid nanoparticles for the food sector [18].
Currently, two kinds of sensitive bio-active food ingredients have been successfully encapsulated into SLN and NLC delivery systems—β-carotene and lutein. For instance, Tripplet et al have investigated the effects of size distribution, stability, drug loading and drug release of β-carotene from stearic acid nanoparticles [19]. In the meantime, a complementary study demonstrated the suitability of the SLN vehicle for stabilizing and protecting carotene from degradation by formulating solid lipid nanoparticles into stearyl ferulate [20]. The ability of NLCs based on caprylic/capric triglycerides (Miglyol) to protect and deliver the lutein via oral and topical routes has been underlined by two recent studies [20, 21].
Although the impact of these lipid nanoparticles on the nutraceutical delivery is evident, no investigations have been conducted yet on phytosterol encapsulation. From the phytosterol class, β-sitosterol is the most common bio-active nutraceutical with multiple metabolic activities. Several in vitro and in vivo studies have confirmed the beneficial therapeutic potentials of β-sitosterol [23]. A review of 19 clinical studies reported that phytosterols decreased serum concentrations of total cholesterol by 5–23%, and of LDL cholesterol by 7–29% [24]. β-sitosterol isolated from various plants promotes apoptosis [25], decreases the in vitro free radical generation [26] and inhibits cancer cell proliferation with no cytotoxic effect to noncancerous cells [27].
In this context, our work aimed to broaden the existing class of delivery systems suitable for food active ingredient transport, by means of developing new, stable and efficiently bio-active lipid nanocarriers that show antioxidant properties and are able to offer an appropriate sustained release of sitosterol. The main advantage of this research is associated with the use of some unexplored natural oils such as a complex natural oil with vegetal provenance (grape seed oil), a mixture of ω-3 and ω-6 fatty acids from fish oil and an individual oil extracted from shark liver (squalene) for bio-active NLC preparation. Apart from being the liquid lipid required at the formation of the complex lipid matrix, these natural oils provide broad pharmacological activities and therapeutic potentials [28, 29]. In previous research our group reported a preliminary study on the beneficial effects of two natural oils (squalene and grape seed oil) in the formation of biocompatible lipid nanocarriers loaded with carotene [30]. Effective antibacterial and antioxidant properties have been observed in this previous work, but no study on the nutraceutical release has been discussed [30].
In order to extend the previous research and determine further the in vitro behaviour of the lipid nanocarriers prepared with different natural oils, our primary objective was initially to investigate and optimize the synthesis of bio-active NLCs as a safe and protective formulation for β-sitosterol, by using two experimental protocols and by exploring the potential of these natural antioxidant oils. The relevant combinations of biological solid lipids and liquid lipids derived from natural products with food grade surfactants/co-surfactant (polysorbate 20 and 80, lecithin, block copolymer) have been used for bio-active nanocarrier synthesis and β-sitosterol encapsulation. Moreover, the influence of an additionally natural antioxidant (green tea extract) has also been included in the current research. Another important aim of the study was to determine the effect of grape seed oil, fish oil and squalene on the efficacy of the novel nanocarriers loaded with β-sitosterol or β-sitosterol and green tea extract to scavenge the dangerous free O2⋅- and OH radicals, since no previous investigation had been successful in this area. In addition—for the first time—the optimized combinations of bio-active nanocarriers prepared with grape seed oil and squalene have been explored in terms of their ability and performance to assure a sustained release of β-sitosterol into a simulated biologic fluid.
2. Experimental details
2.1. Materials
β-sitosterol (>96% purity) was supplied by AppliChem GmbH (Germany), Polyoxyethylenesorbitan monooleate (Tween 80), polyoxyethylenesorbitan monolaurate (Tween 20), dimethylsulfoxide and hydrogen peroxide were obtained from Merck (Germany); Synperonic F68 (block copolymer of polyethylene and polypropylene glycol), l-α-phosphatidylcholine (Lecithin) and Tris[hydroxymethyl]aminomethane (Luminol) were purchased from Sigma Aldrich Chemie GmbH. The natural oils, squalene (Sq) was purchased from Merck, grape seed oil (GSO) (with a fatty acid content of 59% linoleic, oleic, palmitic and stearic acids) was from Manicos SRL (Romania) and Menhaden fish oil (FO) was from Sigma Aldrich. n-hexadecyl palmitate (CP) was obtained from Acros Organics (USA) and glyceril stearate (GS) from Cognis GmbH. All other chemicals used were of analytical grade.
2.2. Synthesis procedure for sitosterol-bio-active nanostructured lipid carriers
The free- and loaded bio-active NLCs were obtained by using the melting emulsification coupled with the high shear homogenization technique described by authors elsewhere [30, 31]. In this study, two comparative protocols were tested: protocol (1) the NLCs loaded with sitosterol were obtained by adding a sitosterol solution (0.3 g sitosterol dissolved in 5 ml chloroform) to the melted lipid phase and maintaining it for five minutes before mixing with the aqueous surfactant phase; protocol (2) the NLCs loaded with sitosterol and green tea extract (GTE) have been obtained by first dissolving 0.03 g green tea extract into the lipid phase, followed by adding 0.27 g of sitosterol.
All NLC formulations have been prepared starting from an initial concentration of 10% lipid phase consisting of a mixture of solid lipids (GS, CP) and liquid lipids (Sq, GSO or FO), in two weight ratio (8:2 for NLC-S1–S5, and 7:3 for NLC-S6–S14). This lipid phase was heated to a temperature of 85 °C and then dispersed in a hot surfactant aqueous solution heated at the same temperature. The aqueous solution of 3% (w/v) surfactants consisted of a mixture of Tween 20 or 80:Lecithin:Synperonic F68 = 1:0.25:0.25. The initial composition of each NLC formulation is presented in table 1. In a further stage, the different pre-emulsions obtained by mixing of organic and aqueous phases and kept for 2 h at 85 °C, were submitted to an external mechanical energy by high shear homogenization with a Lab rotor–stator homogenizer (High-Shear Homogenizer SC 250 type; 0–30.000 rpm; power of 250 W, PRO Scientific, USA), by applying 25 000 rpm for 5 min. The obtained emulsion was allowed to cool slowly at room temperature, with the formation of NLC dispersions loaded with β-sitosterol/β-sitosterol and green tea extract. In order to remove the water excess and obtain powders of loaded-NLCs, the nanoparticle dispersions were submitted to a lyophilization process, by using Alpha 1–2 LD Freeze Dry System equipment (Martin Christ Gefriertrocknungsanlagen GmbH, Osterode am Harz, Germany). The dispersions were first frozen at −25 °C for 24 h and after that they were kept at −55 °C for a period of 72 h.
Table 1. The physico-chemical characterization of sitosterol-loaded bio-active nanocarriers.
Formulation | Cbio active compound | Main surfactanta/Natural oilb | Zav (nm) | PDI | ZP (mV) |
---|---|---|---|---|---|
NLC-S1 | 1% sitosterol | Tw 20/20%Sq | 177.9 ± 3.164 | 0.225 ± 0.003 | −46.8 ± 0.586 |
NLC-S2 | 1% sitosterol | Tw 20/20%GSO | 189.1 ± 6.186 | 0.243 ± 0.011 | −47.1 ± 1.420 |
NLC-S3 | 1% sitosterol | Tw80/20%Sq | 251.3 ± 5.260 | 0.267 ± 0.013 | −45.8 ± 0.777 |
NLC-S4 | 1% sitosterol | Tw 80/20%GSO | 258.0 ± 6.438 | 0.315 ± 0.049 | −38.1 ± 1.150 |
NLC-S5 | 1% sitosterol | Tw 20/30%Sq | 183.0 ± 4.535 | 0.244 ± 0.011 | −52.4 ± 2.740 |
NLC-S6 | 1% sitosterol | Tw20/30%GSO | 184.4 ± 4.901 | 0.241 ± 0.006 | −52.2 ± 2.100 |
NLC-S7 | 1% sitosterol | Tw 80/30%Sq | 234.3 ± 4.398 | 0.249 ± 0.007 | −48.5 ± 1.69 |
NLC-S8 | 1% sitosterol | Tw 80/30%GSO | 258.5 ± 3.239 | 0.380 ± 0.018 | −38.7 ± 0.709 |
NLC-S9 | 1% sitosterol | Tw 80/30%FO | 236.8 ± 1.539 | 0.226 ± 0.028 | −48.2 ± 0.751 |
NLC-S10 | 0.9% sitosterol + 0.1% GTE | Tw 80/30%FO | 222.0 ± 1.200 | 0.233 ± 0.005 | −44.1 ± 1.420 |
NLC-S11 | 0.9% sitosterol + 0.1% GTE | Tw 20/30%Sq | 180.3 ± 6.978 | 0.203 ± 0.008 | −42.9 ± 0.520 |
NLC-S12 | 0.9% sitosterol + 0.1% GTE | Tw 80/30%Sq | 187.2 ± 0.800 | 0.249 ± 0.004 | −38.2 ± 0.902 |
NLC-S13 | 0.9% sitosterol + 0.1% GTE | Tw 20/30%GSO | 162.8 ± 0.585 | 0.158 ± 0.007 | −43.5 ± 0.850 |
NLC-S14 | 0.9% sitosterol + 0.1% GTE | Tw 80/30%GSO | 202.4 ± 1.172 | 0.223 ± 0.012 | −36.7 ± 0.985 |
aTo the main non-ionic surfactant, a mixture of lecithin and block copolymer was used, which represents a total of 3% surfactant mixtures;. bIn all formulations a composition of 10% lipid mixture (GS/CP/Sq or GSO or FO) was used.
2.3. Determination of average diameters and polydispersity index of sitosterol-encapsulated bio-active NLCs
The particle size parameters of lipid nanoparticles given by the hydrodynamic diameters, zaverage (the particle diameter plus the double layer thickness) and polydispersity index, PDI of each NLC dispersion were determined by using the dynamic light scattering (DLS) technique (Zetasizer Nano ZS, Malvern Instruments Ltd, UK), at scattering angles of 90° and 25 °C. The PDI is a measure of the breadth of size distribution derived from the cumulative analysis of DLS data. It has a value between 0 and 1. A PDI of 1 indicates large variations in particle size, while a value close to 0 indicates a monodisperse particle population. Dispersions were analysed after appropriate dilution with deionized water to an adequate scattering intensity prior to the measurement. The particle size analysis data were evaluated using the intensity distribution. The average diameters (based on the Stokes–Einstein equation) and PDI were calculated from the three individual measurements. Thus, the mean value ± standard deviation was calculated and reported.
2.4. Stability of sitosterol-NLCs zeta potential determinations
The measurement of electrokinetic potential (ξ) used to assess the charge stability of all NLCs systems was realized by applying an electric field across the analysed aqueous dispersion using the appropriate accessory of the Zetasizer Nano ZS (Malvern Instruments Ltd, UK). Before zeta potential measurements, all the samples were adjusted with a 0.9% NaCl solution in order to correct the conductivity of aqueous dispersions of NLCs at a value of about 55 μS cm−1. All measurements were performed in triplicate.
2.5. Transmission electronic microscopy analysis
The morphology and size of sitosterol-NLCs were examined using a transmission electron microscope (Philips 208 S, Netherlands). The loaded β-sitosterol-lipid nanocarriers were diluted with ultrapure water, in a ratio of 1:50. A drop of the diluted β-sitosterol-NLC solution was placed onto a carbon-coated copper film, kept for 15 min (to allow some of the particles to adhere to the carbon substrate) and then viewed and photographed.
2.6. Percentage of sitosterol loaded into bio-active NLCs
For investigation of entrapment efficiency (EE), the free-/unloaded-sitosterol concentration (which was not entrapped inside the nanocarriers) was determined by using HPLC analysis. Briefly, 0.05 g lyophilized sitosterol-NLCs and 1 ml methanol were mixed in a centrifuge tube and then centrifuged at 15 000 rpm for 25 min (Sigma 2K15, Germany). The collected supernatant containing the free-/unloaded-sitosterol was transferred into a certain volume of methanol. The resulting solution was subjected to HPLC analysis. All samples were analysed using a Jasco liquid chromatograph equipped with a Nucleosil C18 column (5 μm,25 × 0.4 mm) and a UV detector at λmax = 210 nm. The mobile phase was composed of methanol/water (98:2, v/v) and the flow rate was 1 ml min−1. The supernatants and standard samples were properly diluted in methanol before HPLC injection. The per cent of loaded β-sitosterol has been calculated from the concentration (C) relation:

2.7. Differential scanning calorimetry
In order to investigate the changes in the crystalline states of the lipid matrix, the lyophilized β-sitosterol-NLCs, free-NLCs and raw lipid materials were studied by differential scanning calorimetry (DSC). The samples (10 mg) were weighed into standard alumina pans. An empty pan was used as reference. Samples were heated at a scanning rate of 5 °C min−1 over a temperature range between 30 and 100 °C. Thermograms were recorded with a differential scanning calorimeter Jupiter, STA 449C (Erich Netzsch GmbH & Co. Holding KG, Selb, Germany).
2.8. In vitro determination of antioxidant activity
The in vitro antioxidant activity of pure sitosterol, free-NLCs and NLCs loaded with β-sitosterol/β-sitosterol and GTE has been determined by the chemiluminescence method (CL) using a Chemiluminometer Turner Design TD 20/20, USA. A cyclic hydrazide (luminol) has been used as a light amplifying substance which emits light when oxidized and is converted into an excited aminophthalate ion in the presence of oxidizing species such as superoxide ( ⋅O2), hydrogen peroxide (H2O2), the hydroxyl radical ( ⋅OH) and singlet oxygen (1O2). The luminol increases the detection sensitivity of the activated oxygen species in a sample. As a generator system for free radicals H2O2 has been used in TRIS-HCl buffer solution (pH = 8.6). Concentrations of 66.5 μM sitosterol were prepared. The antioxidant activity (percentage of scavenging of free radicals) was calculated by using the relation:
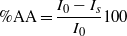
where I0 = themaximum CL for the standard (the standard is composed of a mixture of 50 μl hydrogen peroxide (10−5 M),200 μl luminol (10−5 M) and 750 μl TRIS-HCl buffer solution) at t = 5 s; Is = themaximum CL for the sample at t = 5 s.
2.9. In vitro release study of sitosterol from developed bio-active NLCs
The release patterns of β-sitosterol from the lipid nanocarriers were performed on an in vitro modified Franz diffusion cell (25 mm in diameter, Hanson Research Corporation, USA). The cell consisted of donor and receptor chambers between which a cellulose membrane (Teknokroma, Spain) of 0.45 μm average pore size was positioned. A total volume of 300 μl of sitosterol-bio-active NLC dispersion was applied to the donor compartment. The receptor fluid (6 ml) consisted of a solution of phosphate-buffered saline (pH = 5.5):ethanol. The addition of 30% ethanol in the receptor medium was chosen because of the insufficient solubility of sitosterol in aqueous buffer. Each receptor chamber contained a magnetic stirrer to maintain the homogeneity of buffer during the 72 h of experiment. The temperature of the receptor chamber was controlled at 37 °C by a water circulator. At specified intervals, 300 μl of the receptor medium were withdrawn and the same volume of fresh phosphate buffer solution was added. The sitosterol release concentration was analysed by HPLC using the same protocol described at section 2.6.
3. Results and discussion
Knowing the precarious stability of β-sitosterol, its encapsulation into usually lipid nanoparticles does not assure their stabilization. For this reason, in this study a complex mixture of different lipids has been used in order to create an appropriate lipid matrix for sitosterol stabilization. Due to the great interest in natural antioxidants, especially of plant origin, a complex natural oil with vegetal provenance (GSO), one from animal source (mixture of ω-3 and ω-6 fatty acids from fish oil) and an individual oil extracted from shark liver (Sq) have been selected for bio-active NLC preparation.
3.1. The dimensional and morphologic characterization of sitosterol-NLCs
The first stage of the study of sitosterol encapsulation into bio-active lipid particles involved the evaluation of size distribution and PDI of sitosterol loaded-NLCs as a function of natural oil and mainly non-ionic surfactant type. Some comparative experimental protocols have been tested for keeping the structural integrity of lipophyl compounds: (i) the formation of a bio-active nanomatrix based on individual Sq or a complex vegetal mixture of GSO) (ii) the use of a stronger antioxidant oil (e.g. a mixture of ω-3 and ω-6 fatty acids from Menhaden fish); (iii) the introduction of a supplementary antioxidant from a vegetal source—GTE. In this respect, different aqueous NLC dispersions loaded with 1% sitosterol or a mixture of sitosterol with GTE and prepared with two natural oils and the main non-ionic surfactants have been analysed by the DLS technique. A first observation drawn from DLS measurements has revealed that the bio-active lipid nanocarriers prepared by both experimental protocols present a narrow size distribution and good polydispersity index (figures 1(a) and (b)).
Figure 1. Size and morphologic evaluation of β-sitosterol-NLCs. (a) The effect of natural oil composition, by the DLS technique. (b) The influence of natural oil type and of GTE, by the DLS technique.
Download figure:
Standard imageFor the first experimental protocol, the average diameters of sitosterol-loaded-NLCs have varied from 177 to 260 nm and the PDI was less then 0.25 for almost all the samples (figure 1(a)). It was found that, the samples prepared in the Tween 80/ Lecithin/Block copolymer system led to bigger bio-active NLCs, while in the case of the Tween 20 system, smaller NLCs have been obtained (figure 1(a)), for both kinds of natural oils. For instance, average diameters of 177.9 nm with Pdl = 0.225 (NLC-S1) and 189.1 nm with Pdl = 0.243 (NLC-S2), have been obtained for a composition of 20% Sq/GSO. In the case of a higher composition of natural oil (30% Sq/GSO), smaller bio-active NLCs have been obtained (e.g. 183.0 nm, Pdl = 0.244 for NLC-S5 and 184.4 nm, Pdl = 0.241, for NLC-S6, respectively). The better behaviour of Tween 20 than Tween 80 can be associated with the presumption that a surfactant with a short tail is preferred, bearing in mind the complex structure of both natural oils. The possible steric hinderance may also influence the accommodation of sitosterol, leading to higher diameters in the case of Tween 80.
The influence of natural oil existent in the lipid matrix on the main diameters of final loaded-NLCs was studied by preparation of nanocarriers with two percentage amounts in the lipid mixture, while other ingredients were kept constant. As shown in figure 1(a), variation of natural oil from 20% to 30% had no significant influence on the particle size and PDI of bio-active NLCs, only a slight decrease of average diameters of a few nanometres was observed in the case of Sq as compared to the use of a complex mixture from the GSO. These results encourage the use of vegetable oils instead of Sq isolated from fish oil. Therefore, a composition of 30% natural oil was considered optimum for the second experimental protocol, taking into consideration the small difference between Zaverage of NLCs prepared with 20 and 30% Sq or GSO. Moreover, the use of a higher content of natural oil is desired in order to supply appropriate antioxidant properties and a more disordered lipid matrix.
Interesting behaviour was observed by using the second experimental protocol (figure 1(b)), where the presence of an additional natural antioxidant—GTE—generated a notable effect on the particle size of bio-active NLCs. The obtained results have shown that the use of GTE leads to a significant decrease of Zav, as compared with the samples prepared without GTE (e.g. NLC-S5 versus NLC-S11, NLC-S6 versus NLC-S13, NLC-S7 versus NLC-S12, NLC-S8 versus NLC-S14). Among the bio-active NLCs prepared with GTE, the smaller lipid nanocarriers have been obtained for the 0.9%Sitosterol/0.1%GTE/3%Tween 20/30%Sq or GSO systems, with average diameters of 180.3 nm, Pdl = 0.203 (for NLC-S11) and 162.8 nm, Pdl = 0.158 for NLC-S13, respectively, which indicate a narrow distribution of bio-active nanocarrier populations.
There are several explanations for these unexpected results. Firstly, the longer chain of Tween 80 allows a better accommodation of polyphenols from GTE and as a result lower diameters are obtained. Secondly, as can be seen later in the stability evaluation of prepared bio-active NLC-nanodispersions, the shell formed by the surfactant mixture is affected by the presence of GTE, meaning the existence of a rearrangement of surfactant shell with a better assembling due to a possible interaction of zwitterionic surfactant with the polyphenols from GTE. A modification of the hydrophyl/lipophyl balance caused by the high content of hydroxyl groups from the polyphenol structure of GTE, could also have an important influence on the main diameters of lipid nanocarriers.
The results obtained by the DLS technique have been confirmed by microscopy analysis. The TEM images of four optimized sitosterol-loaded—bio-active—NLCs are presented in figure 2. It can be observed that whatever the type of natural oil used (GSO or Sq), the particles have a spherical shape and no irregular crystallization with needle crystals is visible. This last aspect underlines the high content in the least ordered crystal structure (α-form) of the lipid phase. According to literature data, a β-modification of the lipid matrix induces the appearance of a typical elongated, needle-shape characteristic to a more ordered structure [32]. This observation is also confirmed by the DSC analysis.
Figure 2. TEM image for optimized sitosterol-NLCs prepared with both protocols.
Download figure:
Standard imageThe general observation detached by comparing the TEM images of sitosterol-NLCs prepared with both experimental protocols is associated with a visible lower size of lipid nanocarriers loaded with both active compounds (sitosterol and GTE).
3.2. Stability evaluation of prepared sitosterol lipid nanocarriers
Since an absolute large negative or positive zeta potential is required for colloidal dispersion stability and taking into consideration that the developed NLCs mainly based on natural oils are innovative lipid systems, the evaluation of electric charge on the particle surface represents a valuable prerequisite. The measuring of the electrostatic potential (ξ) of sitosterol-loaded bio-active nanocarriers showed no remarkable changes in the average zeta potential of these samples (table 1, figure 3). All the developed bio-active NLCs manifest an excellent physical stability, with electrokinetic potential values ranging between −36.7 and −52.4 mV. As expected, the samples prepared with Tween 80/Lecithin/Poloxamer/GSO and the first protocol present slightly increased ξ values (around −38 mV), while the bio-active NLC prepared in the Tween 20 system and both Sq and GSO provide a strong electrical field around the particles that leads to values of −52 mV. All these results demonstrated that the combination of steric and electrostatic stabilization provided by the surfactant mixtures in correlation with the natural oils will avoid the nanocarriers aggregation in time. It is worthwhile noting that the zeta potential values of the sitosterol-NLCs prepared with the GTE (second experimental protocol) showed less negative values than those of nanocarriers prepared without GTE (e.g. from −52 mV for NLC-S5 to −42.9 mV for NLC-S11 and from −48.5 mV for NLC-S7 to −38.2 mV for NLC-S12, respectively). This trend of the zeta potential as a function of GTE presence should be logically attributed to the surface association of multiple OH-groups from GTE with the ion charged lecithin. This surface association process is assumed to be driven by the electrostatic interaction.
Figure 3. Evaluation of the sitosterol-bio-active NLC stability by zeta potential distribution.
Download figure:
Standard image3.3. Evaluation of lipid crystallinity and the effect of natural oil type on the entrapment efficiency of sitosterol into bio-active NLCs
The evaluation of the crystalline structure of lipid nanocarriers before and after loading with sitosterol has been achieved by DSC. Thermal analysis was performed 30 days after sample preparation and the results are illustrated in figure 4.
Figure 4. DSC curves for the lyophilized sitosterol-NLCs, compared with free-NLCs: (a) NLCs prepared with FO; (b) NLCs prepared with Sq; (c) NLCs prepared with GSO.
Download figure:
Standard imageBy a brief analysis of DSC thermograms of sitosterol-NLCs it could be observed that the loaded bio-active nanocarriers prepared with different surfactant mixtures and heterogeneous natural oils presented similar endotherm behaviour in the close temperature range, indicating that the natural oil type did not yield to different modifications of the lipid network. As was previously demonstrated by TEM analysis, the spherical shape of developed bio-active NLCs underlines a highly disordered crystalline structure of all samples, by means of the presence of only α-modification of the lipid phase. This represents an advantage, bearing in mind that the obtaining of ordered crystalline structures results in undesired sitosterol expulsion.
The comparative endotherm curves obtained for all bio-active NLC-loaded with sitosterol have evidenced the following aspects.
- A decline in the melting point and melting enthalpy in almost all the loaded-NLCs, compared with free-NLCs. This behaviour clearly indicates the effect of active components on the lipid crystallization patterns (figure 4). The incorporation of both sitosterol and GTE has led to a decrease in the crystalline arrangement. Moreover, according to some literature data a decrease in melting point is a result of a large surface-to-volume ratio—in particular a lower size of particles [33]. These observations could be easily correlated with the DLS results. The lower melting point corresponds to lower Z average (e.g. NLC-S9 versus NLC-S10 and NLC-S13 versus NLC-S14).
- The use of GSO caused the most important perturbation of the lipid network (the presence of lattice defects/less ordered crystalline network), while the fish oil has not led to an evident broadening of the heating peak (figure 4(a)). This last aspect underlines a more ordered crystalline arrangement with significant effect, as may be seen later, on the entrapment efficiency of sitosterol.
- An interesting result was obtained by comparing the effect of the main non-ionic surfactant on the crystallization pattern of NLCs prepared with individual liquid oil (Sq) and complex natural oil (GSO): (i) NLCs prepared with Sq (figure 4(b)) have led to a similar crystalline arrangement, whatever the type of non-ionic surfactant used; (ii) in the case of NLC prepared with GSO, the main non-ionic surfactant has significantly influenced the crystallization pattern (figure 4(c)).
These differences could be explained starting from a relevant hypothesis based on the structural characteristics of natural oil. Being a strongly lipophyl compound, Sq participates in the formation of a disordered crystalline network, with the same lattice defects (with both non-ionic surfactants), while the complex structure of GSO (fatty acids and bioflavonoids) led to a different lipid arrangement with respect to type Tween 80 or Tween 20 surfactant. From a brief comparison of the DLS–DSC–HPLC results, it could be deduced that the lipid crystalline structure related to the chemical nature of the lipid is a key factor to determine how the sitosterol will be incorporated into the carrier system. It is well known that the lipid forming a highly crystalline state would lead to active compound expulsion, while the lattice defects of the lipid structure offer appropriate spaces to accommodate the active compound. In other words a less ordered arrangement in the lipid structure and increased imperfections suggest high entrapment efficiency. In this study, the HPLC analysis used to quantify the sitosterol entrapment supports all the previous presumptions (figure 5). The higher entrapment efficiency of sitosterol in the bio-active NLCs has been obtained for the nanocarriers prepared with Sq and both kinds of non-ionic surfactants (EE = 87%), followed by NLC-S10 prepared with FO (EE = 83%) and NLC-S14 prepared with GSO (EE = 82%) and Tween 80 as the main non-ionic surfactant (figure 5). The high entrapment efficiency, over 82%, indicated an appropriate accommodation of the sitosterol inside the imperfections of the complex lipid core of the developed NLCs.
Figure 5. Effects of individual and complex natural oils on the entrapment efficiency of β-sitosterol into bio-active NLCs.
Download figure:
Standard imageThe lowest EE% has been observed for NLC-S9 prepared with FO (72.4%). This low EE% could be justified by a good compatibility between the fish oil and solid lipids used for obtaining the solid core, thus by its tendency to form a more ordered lipid network, and as a result less space is available to accommodate the sitosterol. Moreover, the ordered crystalline matrix was confirmed by the allure of an endotherm peak that does not show a significant perturbation after sitosterol incorporation (figure 4(a)). Anyway, by the association of FO with the GTE, a rearrangement of the crystalline network, i.e. a transition of lipid nanocarriers to a less ordered solid state, was accompanied by a significant increase of EE% from 72% (without GTE) towards 83% (with GTE). This increase of EE% could be easily correlated with the decrease of endotherm peak observed in figure 4(a).
3.4. In vitro determination of the antioxidant activity of sitosterol-bio-active NLCs prepared with various natural oils
Scavenging reactive oxygen species (ROS) by antioxidant activity is important in preventing potential damage to cellular components, including DNA, proteins and lipids. Oxidative damage can cause major events, sometimes leading to carcinogenesis. Antioxidants have been used to inhibit apoptosis because apoptosis appeared initially to be mediated by oxidative stress [34]. In this respect, the development of functional nanostructures with nutraceutical content and improved antioxidant properties is one of the major challenges in the food industry that will be significantly enhanced by the development of a combined approach of nanotechnology with the main specific properties of some bio-active natural oils and lipophyl nutraceuticals. Starting from this idea, this part of the research involved a comparative determination of antioxidant activity (AA%) of sitosterol bio-active NLCs as a function of the type of host lipid network and the presence/absence of an additional natural antioxidant—GTE.
From figure 6, it may be observed that the bio-active sitosterol manifests a low ability to scavenge the free radicals. At a concentration of 66.5 μM, it scavenges only 36.5% of oxygen derived free radicals, generated in the chemiluminescence system. This low AA% is significantly changed by sitosterol encapsulation into different bio-active NLCs.
Figure 6. In vitro determination of antioxidant activity of native β-sitosterol, free- and loaded bio-active-NLCs, as a function of preparation procedure, type of natural oil and main surfactant.
Download figure:
Standard imageThe AA% of encapsulated sitosterol was amplified compared to that of pure sitosterol (figure 6), the highest difference being obtained in the case of a lipid matrix formed with Sq and GSO. The explanation for this behaviour has been initially associated with the double size and encapsulation effects. This presumption was removed by comparing the AA% of the free-NLCs. Whatever the type of natural oil, all the free-nanocarriers present good antioxidant properties (e.g. free-NLC prepared with Sq present a value of 52%, those prepared with GSO—62%, while the formation of a lipid matrix with FO has led to a value of 78%). However, by studying the antioxidant behaviour of the empty-NLCs with those of sitosterol loaded-NLCs (first experimental protocol), a slight increase of AA% was observed in the last samples (e.g. the sitosterol-NLCs prepared with Sq manifest an antioxidant activity of ∼75%, while sitosterol-NLCs prepared with GSO had a value of about 70%).
The results obtained in the case of the second experimental protocol showed that the NLCs which co-encapsulate sitosterol and GTE present a net superior antioxidant activity, with a capacity to scavenge free-oxygen radicals between 90.3% and 92.4% (figure 6), whatever the non-ionic surfactant.
The difference between loaded bio-active NLCs prepared with different experimental protocols could be associated with a combined effect: (1) the size effect of encapsulated β-sitosterol, in combination with its accommodation inside the different lipid cores; (2) the performance of complex lipid core, meaning the appearance of a synergistic effect between lipid components. The fatty acids from GSO and FO together with the phenolic compounds from GTE can act in synergy. This means the existence of many free radical quenching sites able to neutralize the free radicals.
Unexpected results have been observed by modification of the lipid core with a mixture of ω-3 and ω-6 fatty acids from FO. In this case, the presence of GTE shows no significant effect on the antioxidant properties (∼90% for sitosterol NLC without GTE, 91% for those with GTE, as compare with free-NLC—78%). The relevant hypothesis could be associated with the β-sitosterol content.
3.5. The sustained release properties of the new bio-active lipid nanocarriers loaded with sitosterol
The in vitro sitosterol release study on the new bio-active nanocarriers has been investigated by using Franz diffusion cells, often used to evaluate in vitro drug release. The experimental methodology fully explains the important relationship between the lipid composition (structure of liquid and solid lipids) and the degree of nutraceutical release. Four kinds of vehicles including bio-active-NLC prepared with GSO and Sq and their related nanoemulsions (NEs) were used to entrap the sitosterol and evaluate the in vitro release profile. The preparation of NEs was similar to those of NLCs except that Miglyol oil was used to replace the solid lipids in NLCs.
The release profiles presented in figure 7 shows that whatever the type of lipid core (e.g. a solid core, as in the case of new bio-active NLCs, or a liquid core as in the case of NEs), an initial burst release (often encountered in other related systems) did not appear during the first hour of release experiments. The results of the in vitro release studies showed that a certain amount of sitosterol was released from sitosterol-loaded nanoemulsions after 2 h, while in the case of sitosterol-loaded NLCs the release begins only after 4 h.
Figure 7. In vitro release profiles of β-sitosterol from optimized bio-active NLCs in comparison with nanoemulsions, as a function of type of natural oil.
Download figure:
Standard imageThe release rate of sitosterol in the phosphate-buffered saline increased in the order NE–Sq, NLC–Sq, NE–GSO, NLC–GSO. As may be seen from figure 7, this sustained release observed for all the tested carriers is maintained for an interval of 72 h. The low and gradual release of sitosterol from NLCs and NEs could be justified by the remarkable affinity of sitosterol to the complex lipid mixture. The presence of liquid reservoirs of GSO and Sq inside the solid core lead to a high tendency of sitosterol to remain entrapped for a longer time inside the network lattice.
The release of sitosterol from NLC was significantly slower than that of sitosterol loaded-NE (figure 7). In the case of vehicles prepared with GSO the sitosterol release reaches 45% for NLC and 61% for NE, after 48 h of release. The presence of Sq in the lipid core provided a higher sitosterol release (e.g. 75% for NLC and 91% for NE). The droplet vehicles of NLCs provided larger total surface areas for sitosterol diffusion compared to nanoemulsions. Additionally, the solid shell in the NLCs protects and immobilizes the sitosterol in the core of the Sq/GSO droplets. Furthermore, in the case of NE there is a fast rate of hydrolytic degradation (ester reactions) as compare to the NLCs.
With respect to the type of bio-active NLCs, the nanocarriers prepared with GSO were found to have more-sustained release compared to those prepared with Sq (e.g. NLCs prepared with GSO release 55% after 52 h, while those prepared with Sq release 85%). This effect of lipid composition on the sitosterol release percentage from NLCs could be first of all related to the different inner/core structure of NLCs and consequently to the different crystallinity of the particle matrix, as discussed above. Secondly, the slowest release of sitosterol from NLC–GSO could be assessed from the manner of the sitosterol incorporation in the particle matrix. If the sitosterol is enriched in the particle core (drug core–shell model), release is prolonged. In other words, the difference between the two bio-active NLCs could be also attributed to the longest diffusion distance from the donor chamber to the receptor buffer medium.
4. Conclusion
New bio-active lipid nanocarriers based on natural oils that present significant antioxidant activity have been developed and applied for encapsulation, stabilization and sustained release of β-sitosterol. The developed β-sitosterol-NLCs were physico-chemically characterized for their particle size, in relation to their stability against aggregation and to their structural modification after β-sitosterol encapsulation. The smaller lipid nanocarriers have been obtained by a co-encapsulation of β-sitosterol and GTE, with average diameters of 180 nm/Pdl = 0.203, for NLC-S11 and 162.8 nm/Pdl = 0.158 for NLC-S13, respectively, which indicate a narrow size distribution of bio-active nanocarrier population. Stability evaluation of all loaded-bio-active NLCs has shown an excellent physical stability with zeta potential values ranging between −36 and −52 mV. The less ordered arrangement of bio-active lipid core, emphasized by the scanning calorimetry results, doubled by the high entrapment efficiencies of 72–87% β-sitosterol, encourage the use of natural oil from vegetable source (e.g. grape seed oil) instead of other synthetic lipids.
The presence of a supplementary natural antioxidant, even in a low concentration, has generated a notable effect on the particle size of bio-active NLCs and also on the antioxidant activity. The obtained results clearly indicate that the use of GTE has led to a significant decrease of Zav, while the antioxidant properties have been significantly enhanced.
All types of β-sitosterol loaded-NLCs have the potential to develop a high blocking action of the chain reactions in vitro generated in a chemiluminescence system. At low concentration, the native β-sitosterol manifests a low ability to scavenge the free radicals (e.g. it scavenges only 36.5% of oxygen derived free radicals), while the antioxidant capacity of loaded-bio-active NLCs has reached 92%. Another advantage of this study is associated with the main feature of the developed bio-active NLCs based on grape seed oil and squalene to manifest a better sitosterol-sustained release behaviour as compared to their related nanoemulsions.
By coupling both in vitro results, i.e. the enhanced antioxidant activity and the ability of the new nanocarriers to allow an appropriate sustained release of nutraceuticals, this study underlines the value of natural oils which could be of considerable interest for the development of functional foods with a high spectrum of applicability in the food sector. The formulation of β-sitosterol into colloidal lipid nanocarriers of fat-in-water dispersions could serve to successfully incorporate this nutraceutical into water-dispersible food systems. Beyond the specific case described here, this study provides evidence that these new nanocarriers may allow us to gain specific bio-food features, such as a modified release of any kind of nutraceutical.
Acknowledgment
The authors acknowledge the financial support from the European Social Fund through POSDRU/89/1.5/S/54785 project: 'Postdoctoral Program for Advanced Research in the field of nanomaterials'.