Abstract
For decades the global positioning system (GPS) has been the only space geodetic technique routinely used for inter-continental frequency transfer applications. In the past very long baseline interferometry (VLBI) has also been considered for this purpose and the method's capabilities were studied several times. However, compared to GPS current VLBI technology only provides few observations per hour, thus limiting its potential to improve frequency comparisons. We therefore investigate the effect of combining GPS and VLBI on the observation level in order to draw the maximum benefit from the strength of each individual technique. As a test-bed for our study we use the CONT11 campaign observed in 2011. First we review the frequency transfer performance that can be achieved with independent technique-specific analyses, both with individual software packages and with the multi-technique software c5++. With this analysis approach both techniques, GPS and VLBI, show similar frequency link instabilities at the level of 10−14 to 10−15 (MDEV) on inter-continental baselines for averaging times of one day. Then we use the c5++ software for a combined analysis of GPS and VLBI data on the observation level. We demonstrate that our combination approach leads to small but consistent improvements for frequency transfer of up to 10%, in particular for averaging periods longer than 3000 s.
Export citation and abstract BibTeX RIS

Content from this work may be used under the terms of the Creative Commons Attribution 3.0 licence. Any further distribution of this work must maintain attribution to the author(s) and the title of the work, journal citation and DOI.
1. Introduction
International timekeeping is a combined effort of many national timekeepers that have pushed the tremendous development of time metrology throughout the years [1]. As a major result next-generation optical frequency standards are expected to outperform current microwave atomic clocks by several orders of magnitude and this will likely lead to a redefinition of the SI second [2]. In order to compare and assess such advanced frequency standards it is necessary to develop new and improved methods for inter-continental time and frequency transfer.
Currently operationally used methods like two-way satellite time and frequency transfer (TWSTFT) and the use of global navigation satellite systems (GNSS) such as the global positioning system (GPS) (e.g. [3]) need to be improved in order to be useful for the comparison of next-generation of frequency standards. Methods based on signal transmission via optical fibers seem to be a very promising solution for comparing modern atomic clocks [4]. Network topology and the spatial coverage of fiber cables still restricts this approach although distances of more than 1000 km have been bridged recently [5]. Advanced TWSTFT technology [6] might provide enough precision over inter-continental distances, but depends on the availability of satellite transponders. The future ESA experiment Atomic Clock Ensemble in Space (ACES), which will be operated on-board the International Space Station (ISS), appears to be another promising method that can help to establish highly precise inter-continental timing links [7].
Other suitable space-geodetic techniques are satellite laser ranging (SLR) [8] and Very Long Baseline Interferometry (VLBI). In several studies (e.g. [9]) the potential of VLBI for frequency comparisons has been investigated. These studies generally show that VLBI and GPS provide similar frequency transfer stabilities for averaging periods longer than a few hours. For shorter averaging periods the use of VLBI is limited by its low data sampling rate, which results in a low temporal resolution of the clock estimates. Thus VLBI cannot match the short-term capabilities of GPS.
However, in all prior investigations the VLBI and GPS analyses were carried out with individual and independent software packages. Since these analysis packages use slightly different geophysical models, small inconsistencies are caused that impact the comparison of the results derived from the two techniques. Also the fact that the different software packages often use different statistical analysis approaches complicates these comparisons. Some of the analysis packages are based on a simple least-squares estimation process, whereas others make use of sequential/Kalman filtering methods.
In order to overcome the drawback of low temporal resolution of the VLBI clock estimates, one idea is to combine VLBI and GPS data on the observation level and to evaluate whether such a combined solution benefits from the strengths of the individual techniques. In addition, VLBI and GPS single-technique frequency performances can be better compared against each other and with respect to such a combined solution when all processing is handled by one single software package that ensures consistency of the applied geophysical models and the estimation method. Since such an analysis tool has become available (see section 2.2) the potential of VLBI for frequency transfer will be evaluated rigorously in the following.
2. VLBI for frequency transfer
Given the fact that VLBI stations are equipped with highly precise and short-term stable frequency standards, usually hydrogen masers, comparing these atomic clocks appears to be straightforward. Since the early days of VLBI, several studies have dealt with the topic of applying this technology for time and frequency transfer (e.g. [10]). Also in [11] the use of VLBI for time and frequency metrology is discussed and the potential of this technique is pointed out. However, it has been stated that VLBI systems have several drawbacks that compromise the application of VLBI for such purposes on a routine basis. First, current VLBI systems are not operating continuously. Observation sessions are usually scheduled to last for only 24 h, which prevents frequency comparisons on time scales longer than one day. Second, as most of the cable and electrical path lengths in the VLBI system are not calibrated in an absolute sense and are designed to be variable, this technology cannot directly be utilized for time comparisons. Frequency transfer is still possible on time scales shorter than the variation, estimated to result in a 1 ns variation over a period of a few days.
In order to understand how VLBI can be a potential frequency transfer technique over inter-continental distances one needs to recall the basic principle of this space geodetic technique. As illustrated in figure 1, VLBI telescopes observe signals from extra-galactic radio sources that are recorded and time-tagged by clocks referenced to the local oscillators, typically hydrogen masers. The difference between the arrival times measured with the respective clocks at the stations is determined by cross-correlation of the recorded data. In case of absolutely calibrated cable and instrumental path lengths, the clock difference follows when subtracting the theoretical delay [12]. Thus, VLBI is in principle able to directly determine the differences between clocks at two sites, if the Earth's orientation, the station positions, as well as ionospheric and tropospheric delays are known or simultaneously fitted. Regarding the station positions, it can be stated that station coordinates of geodetic VLBI sites can be modeled with mm-accuracy, which allows using this as a priori information when performing time and frequency transfer. The delay in the ionosphere can be removed by observing at two or more different frequencies. Tropospheric delays depend on the observation direction and state of the atmosphere. They can be handled by performing measurements at different elevation angles and estimating the corresponding zenith delays with the so-called mapping functions as the partial derivatives. Since mapping functions are elevation-dependent but clock differences are independent of the elevation angle, it is possible to separate both quantities in the estimation process. However, as mapping functions can only approximate true meteorological conditions to a certain extent, errors in the estimation of tropospheric parameters might propagate into both clock parameters and station heights when estimated.
Figure 1. Measurement principle of VLBI. Signals from extra-galactic radio sources observed with radio telescopes are recorded and time-tagged using stable frequency standards, e.g. hydrogen masers. Cross-correlation provides the difference between the arrival times at the stations which can be used to derive the clock difference when subtracting the theoretical delay and propagation effects.
Download figure:
Standard image High-resolution image2.1. Clock differences as a by-product of geodetic VLBI analysis
No technique is capable of providing absolute clock parameters at each site. Therefore it is necessary that one clock at one selected station in a VLBI network is kept fixed and all other clocks of the other stations in the network will be related to this reference clock. By doing so, clock differences can be obtained from geodetic post-processing, i.e. the adjustment of the unknown parameters. Since the clock difference parameters need to be estimated together with the other unknowns, one needs to pay attention that neither unmodeled effects bias the clock estimates, nor correlations among the unknowns absorb clock variations in other parameters. Moreover, the parametrization of the clock unknowns has to reflect the physical behaviour of the clock, i.e. providing a temporal sampling which can follow a random walk (in phase) noise process.
2.2. Space geodetic data analysis with c5++
The software package 'c5++' [13] has been developed with the purpose of supporting the combination of space geodetic data from VLBI, GPS and SLR on the observation level and also to allow processing of single-technique solutions. Since c5++ uses consistent geodetic/geophysical models [14] for all space geodetic techniques and all analysis steps, it is guaranteed that differences of the estimated parameters that are derived from e.g. VLBI and GPS do not originate from different models or inconsistent corrections, but are directly related to the performance of each technique. Moreover, the possibility to combine data on the observation level allows to study the impact of combining techniques, with the purpose to improve the target parameters. The concept of combination on the observation level has been successfully demonstrated in [15] and will be applied here (see section 3.3).
3. Frequency transfer during CONT11
CONT11 was a 15 d campaign of continuous VLBI sessions observed september 15–30, 2011. The campaign was scheduled in daily sessions (0–24 UT) so that data or results could easily be combined with other space geodetic techniques over the same time-span. In total 14 VLBI stations participated in the CONT11 campaign, but only 11 of the network stations shared a common frequency standard with a co-located GPS receiver [17].
Furthermore, unfortunately some of the stations were excluded because they experienced technical or observational problems. For example, the stations Badary and Zelenchukskaya experienced strong radio frequency interference (RFI) disturbances in several of the observed frequency bands. The station Tigo Concepción performed rather poorly, mainly due to its small antenna size and several other sites had clock jumps of the VLBI equipment or missing GPS data. Although small antennas, like Tigo, are very attractive as they can be easily deployed at arbitrary sites, technology which was available during CONT11 causes a significant performance degradation that comes with shrinking the antenna diameter w.r.t. the average size of the other VLBI dishes in the network. This drawback is currently being worked on and thought to be overcome by switching to broad-band receiving systems (see section 5). Thus, the further analysis concentrates in the following mainly on the sub-set of 6 of the CONT11 stations shown in figure 2.
Figure 2. Location of the six CONT11 stations that were considered for this study. Only sites were selected where a common frequency standard was connected to both VLBI and GPS equipment and no major technical or observational problems occured. Sites are abbreviated with their IGS name, i.e. Hartebeesthoek (HRAO), Kokee Park (KOKB), Onsala (ONSA), Tsukuba (TSKB), Westford (WES2) and Wettzell (WTZZ).
Download figure:
Standard image High-resolution image3.1. VLBI and GPS analysis with independent software packages
In a first attempt, the complete CONT11 VLBI data set was analysed with the Calc/Solve software [16] following a standard network analysis approach, as described in [17]. Even the previously mentioned 'problematic' stations (section 3) were included in the analysis. The hydrogen maser at Wettzell (Germany) was used as reference clock for the network and relative clock estimates w.r.t. this reference were estimated for all stations as daily second order polynomials with additional continuous spline corrections with a temporal resolution of 20 min. Since the original data correlation and analysis was done in daily batches, the resulting clock estimates were not continuous over day boundaries. To generate continuous series of clock estimates, the daily solutions were fitted together using overlapping estimates, thus removing potential discontinuities at day boundaries.
In parallel, GPS data recorded with the co-located GPS receivers at the CONT11 stations, all connected to the same frequency standard as the corresponding co-located VLBI-system, were analysed with the NRCAN-PPP software [18]. The analysis procedure is described in [17] and gave GPS clock estimates with 60 s temporal resolution for all analysed stations for the whole CONT11 campaign. These time series were differenced with respect to the solution for the GPS receiver at Wettzell (WTZZ) in order to produce time series of relative clock estimates on the network baselines corresponding to the VLBI solution.
The individual time series of clock differences derived from VLBI and GPS were then analysed and modified Allan deviation (MDEV, [19]) values were derived for each baseline in order to estimate the frequency link stabilities. The results show that VLBI and GPS perform similarly with best case one day instabilities on the order of 10−15 [17]. Figure 3 depicts as an example the results for a subset of 5 baselines connecting to Wettzell. GPS and VLBI derived MDEV values are shown in black dots and orange squares, respectively. It is evident that technical problems at Tsukuba affect the stability of the solution. The TSKB receiver lost lock on day 3 of CONT14, thus the GPS-derived MDEV values involving Tsukuba are deteriorated since the technical problems were not accounted for in this first study.
Figure 3. Modified Allan deviation (MDEV) plots for frequency links on 5 baselines connecting to Wettzell, as obtained from GPS (black) and VLBI (orange) single-technique solutions with the software packages NRCAN-PPP and Calc/Solve, respectively. It becomes clear that the loss of lock at the TSKB GPS receiver (see section 3.1), that was not accounted for in this analysis, deteriorates the GPS-performance on the corresponding baseline.
Download figure:
Standard image High-resolution image3.2. VLBI and GPS analysis with c5++
In order to assess both short- and long-term frequency stabilities, the data were processed with c5++ in non-overlapping batch solutions with three consecutive days, i.e. covering a period of 72 h each. Thus, the whole CONT11 campaign could be analysed in five 72 h batches. This was possible since the whole campaign had been re-correlated with a consistent delay model that prevents discontinuities at the day boundaries [20]. As mentioned before, VLBI analysis requires that one station clock in the network is fixed to an arbitrary constant value, which is chosen normally to be zero. Doing so, all estimated clocks in the network represent the clock difference between that station's clock and the clock of the reference site. The clock reference should be assigned to a station which is known to show no clock break during an entire session. Again, as in the previous analysis described in section 3.1, the clock at Wettzell (Germany) was selected as reference. Another characteristic of VLBI analysis relating to clock estimates is the irregular temporal spacing of the original observational data. Depending on antenna and receiver characteristics and the flux density of the observed radio sources, optimized observation schedules require different scan-length and varying idle/slewing times. Moreover, hardware problems or operational problems can lead to larger temporal gaps during which a single station is not observing together with the other network sites. In order to accommodate for these difficulties, which could lead to singularities of the design matrices used in the data analysis, it is common to apply constraints on certain parameters.
For clock estimates, which are parametrized as piece-wise linear offsets (PLO) CL (ti) here, a weak constraint on the rate was used, i.e.

The constraint's weight, i.e. the inverse of the formal error σrate, was chosen so that the clock estimates were smoother than the physical variation of the atomic clock at the VLBI site. If VLBI shall be used for frequency transfer, the proper choice of σrate is very important, in order to guarantee that the estimated frequency instabilities really represent the clock performance and are not dominated by the applied constraints [9]. The constraints themselves and the selection of the temporal resolution of the PLO clock model are other issues that need to be considered properly. A too-high temporal resolution of the PLO model bears the consequence that most of the estimated values are strongly dominated by the choice of the constraint rather than by the observational data.
In addition, zenith wet delays (ZWDs), troposphere gradients and station coordinates need to be estimated in order to obtain clock estimates that are not degraded by the correlations among these parameters. The c5++ software allows to process un-differenced GPS observations with the precise-point positioning (PPP) approach [21, 22], using the same geophysical models as used for VLBI analysis. PPP does not require GPS satellites in common-view and becomes independent of the length of the baselines that are defined by the VLBI network geometry. Thus, even inter-continental frequency links can be established by this analysis method. However, satellite orbits and clocks need to be fixed to IGS final products [23] since such parameters can not be estimated for this study, due to the small number of GPS receivers within the CONT11 network.
Since GPS observations are available with a regular and high sampling rate, clock parameters can be estimated without constraints. Moreover, PPP allows to directly access the behaviour of the station clock and frequency transfer between two stations can be performed by differencing the PPP clock solutions of the two sites. Other parameters like zenith troposphere delays or station coordinates have to be estimated together with the clock model, subject to the same correlations as VLBI.
3.3. Combining VLBI and GPS data on the observation level
Combination on the observation level reflects the concept of using all available geodetic data at a site in order to overcome potential deficits of a single technique. Since co-located instruments often share the same infrastructure (e.g. frequency standards) and are expected to experience the same environmental conditions, in particular the atmospheric conditions, one can estimate parameters related to these influences in a common model which only considers biases between the different techniques. It was demonstrated in [15] that the combination of GPS and VLBI on the observation level leads to a small but significant improvement of the geodetic target parameters and in particular improves the stability of the site coordinate time series. Since troposphere and clock parameters were estimated in [15] by a station-wise model it was obvious to investigate how the combination of these two techniques on the observation level can be utilized for the benefit of frequency transfer. As described in [15], troposphere parameters can be estimated with a common model that considers an offset between GPS and VLBI, caused by the height difference between the VLBI and GPS reference points, resulting in a hydrostatic delay difference which remains very stable over time.
On the other hand, a common clock model can be estimated by combining VLBI and GPS only if one considers that instrumental delays are not stable over time but are influenced by temperature-induced changes in reflections (cable multipath) and electrical lengths; for VLBI these can also depend upon the orientation of the antenna. It was suggested in [15] to estimate these inter-technique delay changes by a PLO model with a temporal resolution that is sufficient for representing at least a diurnal signal. A low temporal resolution, i.e. with a PLO step-width of more than 12 h, bears the risk of not modelling all inter-technique delay changes properly. However, a high temporal resolution reduces the benefit of combining GPS and VLBI since a large fraction of the information provided by the second technique gets absorbed into this parameter. Therefore, the proper choice of the temporal resolution for this crucial parameter is explored in section 4.3.
Another issue that arises from the combination of different observation types is related to the question on how to weight the data of each technique. In order to handle this problem with a proper stochastic model one needs to select an estimation strategy as described in the following.
3.3.1. Variance component estimation.
As the parameter estimation in c5++ relies on a Gauss–Markov model [24] it is possible to implement methods to determine the variance components of different groups of observations. The idea of variance component estimation (VCE) goes back to the beginning of the last century [25] and has seen many improvements and refinements based on different algorithms. Following this concept, it is possible in c5++ to estimate the variance component for VLBI observations, station-based GPS variance components for both GPS code- and carrier phase observations, as well as a component that gives proper weight to the local tie information (see section 3.3.2). In order to realize VCE for parameter adjustment problems with several thousand parameters, the simplified algorithm proposed in [26] has been implemented in c5++ . Thus, one can determine the variance factor of the i-th group of observations by

where vi denotes the residual vector of the prior adjustment and Pi is the corresponding variance-covariance matrix. Together with the degree of freedom ri of the i-th group, one can iteratively determine the variance components while solving the least-squares adjustment problem. Since correlations among the observations are not considered here one can update the variance component parameters after each iteration without the need of maintaining the large variance-covariance matrix as required for the original algorithm described in [25].
3.3.2. Local ties.
Full benefit from co-located space geodetic instruments can only be achieved when the inter-technique 3D vectors (the so-called 'local ties') between the reference points of the individual techniques are known. Usually, site surveys provide these local ties which can then be used to relate the reference points of co-located space geodetic instruments to each other. Doing so, one can assure that two or more co-located space geodetic instruments, i.e. placed together at the same observation site, reveal the same site movement over time. Thus it is crucial that local tie measurements are carried out with utmost high precision and are transformed into the terrestrial reference frame so that they can be applied to connect the different co-located techniques. Local ties are usually provided by the agencies that host the space geodetic instruments and are made public by the ITRF (International Terrestrial Reference Frame) center of the IERS (International Earth Rotation and Reference Frame Service). The c5++ software can use the provided local tie information as an independent observation for combination purposes. A detailed discussion on the weighting of local tie information and its impact of combining two techniques on the observation level is given in [15]. However, since VCE has been implemented recently in c5++, it is now also possible to determine the weights of local tie vectors directly and automatically within the estimation process. Although the realization of such an estimation strategy is straightforward, one needs to consider the fact the there is a huge unbalance between the number of space geodetic observations and the number of local tie vectors. Since the precision of local tie measurements could vary from station to station it would in principle be necessary to solve for a variance component of each of the local ties. However, as this leads to an even further unbalance in the VCE it has been decided to estimate only one single variance component that scales the formal errors of all local ties in the station network.
4. Results
In the following the frequency transfer performance derived from the c5++ analysis using the single technique approach as well as the combined solution approach will be assessed. The frequency stability on baselines of the reduced 6-station CONT11 network is evaluated with the MDEV. Epochs when a GPS receiver lost lock were excluded from the analysis (see section 4.3).
4.1. Single-technique results with c5++
Figure 4 depicts the de-trended clock differences from the single-technique VLBI and GPS c5++ analysis for the third 3 d batch. Only baselines connecting to Wettzell are shown here in order to get an idea about the magnitude and behaviour of the clock differences in the network. A good agreement between both techniques is observed. The same small scale clock variations (see baseline WTZZ-ONSA) as well as large scale patterns (see baseline WTZZ-WES2) can be identified in both single technique results, pointing out the potential of combining both techniques for the purpose of frequency transfer. One can also notice that an observational data gap at the Tsukuba VLBI site causes the estimation to rely on constraints, resulting in a straight line during most of September 21, 2011. This means that in a combined analysis, GPS will bridge the VLBI data-gap and this implicitly support this station which would otherwise strongly rely on the choice of the constraints. Figure 5 depicts the frequency transfer instabilities as obtained from the VLBI and GPS c5++ analysis for all possible links in the reduced CONT11 network (6 stations). The recorded GPS data were decimated from 30 s to a regular sampling rate of 5 min, in order to exactly meet the temporal resolution of the estimated clock model, which has been also set to 5 min (see table 1). VLBI observations were available with a more irregular and sparse temporal sampling which enables us to estimate station clocks only with a temporal resolution of 30 min, given that constraints stabilize the solution in case no data is available over a longer period (see section 3.2). Thus, one needs to take into account that for shorter averaging periods, VLBI and GPS frequency transfer stabilities can not be compared directly. Despite this drawback, it becomes clear that on almost all baselines the individual VLBI and GPS solutions provide the same frequency transfer stability for averaging periods of 4 h or longer. This is in good agreement with the results presented in [17] where the complete CONT11 station network (more than 6 stations) was analysed with two individual analysis software packages applying slightly different processing strategies (see section 3.1). Based on the results from the single technique solutions one can conclude that VLBI reaches a frequency transfer stability similar to GPS for longer averaging periods, but does not allow a sufficiently dense temporal sampling of the clock estimates in order to provide any information for shorter averaging periods. Similarly to the concept presented in [27], where GPS and TWSTFT were combined, one could assume that a combination of GPS and VLBI will lead to a frequency transfer performance that benefits from the strength of both techniques.
Figure 4. Clock differences (after reducing a quadratic trend) as obtained from the third 3 d batch solution for VLBI-only(red), GPS-only(black) and the combined solution(blue). Only baselines connecting to Wettzell are shown here. As for the combined solution, results are shown when inter-technique delay changes are modeled by a constant offset. Diurnal signatures are not evident, except perhaps on the WTZZ-KOKB baseline.
Download figure:
Standard image High-resolution imageFigure 5. Modified Allan deviation (MDEV) plots for frequency links over all baselines in the 6 station CONT11 network, as obtained from the three-daily GPS (black) and VLBI (orange) single-technique solutions with c5++.
Download figure:
Standard image High-resolution imageTable 1. Parameter settings for the VLBI-only, GPS-only and combined GPS-VLBI solutions with c5++ .
Solution | |||
---|---|---|---|
Parameter | VLBI-only | GPS-only | Combined GPS and VLBI |
Clock | 30 min | 5 min | 5 min (+PLO) |
ZWD | 2 h | 2 h | 2 h (+ const offset) |
Gradients | 6 h | 6 h | 6 h |
Note: For the VLBI-only solution, the clock rates were constrained with σc = 5 · 10−14 s s−1 and no-net-rotation (NNR) and no-net-translation (NNT) conditions were applied in order to solve for all station positions. The Tsukuba VLBI site was excluded from the NNR/NNT conditions in order to account for changes in the ITRF2008 position [31] caused by the 2011 earthquake. The Earth orientation parameters (EOP) were fixed in all three solutions to the values of EOP 08 C04 [32]. Inter-technique cable delays were estimated by dedicated piece-wise linear offset (PLO) models with different temporal resolutions (see section 4.3).
4.2. Results from combined analysis with c5++
Combining VLBI and GPS on the observation level is the most natural way to estimate common parameters at geodetic co-location sites. With this approach, biases and technique-specific considerations can be taken into account before the observations are combined directly within the adjustment process. Two of such inter-technique biases are those related to troposphere and clock variability. The former one being mainly caused by different heights of the reference points of co-located instruments, which translate into a hydrostatic delay that can be either modeled or, as done in the present study, estimated as a constant offset during each 72 h batch analysis. The latter offset, hereafter denoted as Δclk(t), is expected to be caused by temperature-induced cable delay variations and can either be estimated as a constant offset or parametrized by a PLO model with sufficient temporal resolution to follow cable delay changes. It is obvious that the choice of this inter-technique delay potentially absorbs any benefit that could result from the estimation of a common clock between the two techniques. A too-short temporal resolution of Δclk(t) bears the risk that a large fraction of valuable information from the second technique is not being reflected in the estimated clock. On the other hand, an insufficient model representation of the mostly diurnal cable length changes potentially degrades the combined solution and the obtained clock parameters. Therefore, different choices for the temporal resolution of the inter-technique cable delay model Δclk(t) have been used and their impact on the frequency transfer stability has been evaluated.
4.3. The impact of the choice for the temporal resolution of the inter-technique cable delay model
In order to evaluate whether the combination of VLBI and GPS leads to an improvement of the frequency transfer stability w.r.t. the GPS-only solution, the ratio
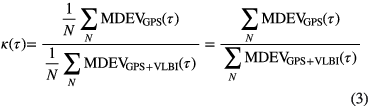
is defined, where N is the number of baselines that are averaged. The ratio κ(τ) describes the average improvement/degradation when combining VLBI with GPS on the observation level for frequency transfer, compared to a GPS-only solution. Since the intervals for the PLO clock model were set to 5 min (see table 1) in both solutions one can compute κ(τ) in a straightforward way. Improvements in the overall frequency transfer performance will then be reflected as κ(τ) > 1, whereas degradations can be recognized when κ(τ) < 1. Since the data were processed in batches of 3 consecutive days one would expect to have N = 5·6·5/2 = 75 baselines when analysing the 15 d long CONT11 campaign with the reduced 6 station network depicted in figure 2. However, since the GPS receiver at TSKB lost lock on the third day of CONT11 and the GPS receiver at HRAO had a similar problem on the 5th day, these two stations were excluded from the first and second 3 d batch solutions, respectively. Thus, the total number of baselines over which κ(τ) can be computed reduces to N = 65. Figure 4 shows how well the clock differences match those obtained from the single-technique GPS PPP solution. VCE helps to determine technique specific weights that integrate VLBI observations in a way which does not degrade the frequency transfer performance, mainly determined by GPS. In order to study this in more detail one needs to evaluate κ(τ) for the different processing options. Figure 6 depicts κ(τ) for solutions with different choices for the temporal resolution of the inter-technique cable delay model Δclk(t). Overall, it can be seen that the combination of VLBI with GPS tends to improve the average frequency transfer stability w.r.t. the GPS-only solution. However, as anticipated in the previous section, the choice of the temporal resolution of Δclk(t) is crucial. The use of an interval length of 1 h for the PLO of Δclk(t) absorbs almost all benefit gained from adding VLBI. On the other hand, it is clearly visible that daily estimates or parametrization as a constant lead to a degradation of the short term stability while improving the long-term stability more than any of the other choices for the temporal resolution of Δclk(t). In general, one can see that VLBI improves the frequency transfer stability for averaging periods between 3000 and 20 000 seconds as well as for periods close to one day. The latter improvement can be explained by the fact that VLBI helps to smooth the jumps introduced by day boundary discontinuities of the used IGS orbit and clock products. However, one needs to consider also the lower significance (higher uncertainty) of the MDEV at the far end of the long averaging period domain. The improvement between 3000 and 30 000 seconds is thought to have its origin in the parametrization of tropospheric estimates, which become more robust against data artifacts, when combining VLBI and GPS. In addition, a temporal resolution of 12 h or longer for Δclk(t) leads to an improvement for averaging periods of 12 h, which might relate to the orbital period of GPS satellites.
Figure 6. Average improvement/degradation of the frequency transfer stability, measured as ratio κ(τ) (see section 4.3), when comparing the combined GPS-VLBI solutions against the GPS-only solution. Results are shown for different temporal resolutions (1 h, 2 h, 3 h, 4 h, 6 h, 8 h, 12 h and 24 h) of the inter-technique delay model as well as for the parametrization with a constant cable delay between the two techniques. Values of κ(τ) > 1 indicate improvement.
Download figure:
Standard image High-resolution imageIn order to assess which baselines benefit from combining the two techniques, κ(τ) was re-sampled to 28 logarithmically equally spaced data points, before the mean for each baseline was computed. This approach allows to derive the average improvement/degradation for a given baseline for which the frequency transfer performance gain is evaluated. Figure 7 depicts these results, displayed as a net chart. It becomes clear that for some baselines the gain of adding VLBI strongly depends on the choice of the temporal resolution of the inter-technique clock delay Δclk(t). It can be concluded that having a half-daily or daily resolution for the PLO model of these delays leads to an improvement on almost all baselines, bearing in mind that this comes mostly from averaging periods longer than a few hours and is accompanied by a degradation in the shorter time domain (see figure 6). It is also worth to mention that there is hardly any improvement on the shortest baseline in the network, i.e. WTZZ-ONSA, which can be explained by the fact that any error introduced by orbit or satellite mismodeling affects the PPP solutions at both sites approximately at the same level and thus cancels out to a large extent when forming the baseline differences and computing the frequency transfer stability over this link. On the other hand, on long intercontinental baselines it can be seen that κ(τ) is always larger than 1, which indicates that VLBI helps to improve these frequency links.
Figure 7. Average improvement/degradation of the frequency transfer stability after re-sampling κ(τ) to 28 logarithmically equally spaced data points for all baselines in the station network. Values of κ(τ) > 1 indicate improvement.
Download figure:
Standard image High-resolution imageWe close this section by noting that use of the Allan Deviation statistic produced similar results and that the question of optimization might be a fruitful subject of further study.
5. Discussion and outlook
VLBI and GPS are two space geodetic techniques operating in the radio frequency domain. GPS is used for time and frequency transfer applications on a routine basis since several years. VLBI, which works on the principle of comparing clock readings over inter-continental distances, has been investigated several times in the past concerning the feasibility to use this technology for frequency transfer. In this study we confirm that VLBI single-technique solutions can provide similar or slightly worse frequency transfer performance as compared to GPS PPP solutions. However, we also demonstrate that the combination of GPS and VLBI on the observation level results in an improvement of the frequency link stability at various averaging periods. On average the combination approach performs consistently better than the GPS-only solution, revealing an improvement of the frequency transfer stability of up to 10% . This leads to the question how VLBI can efficiently contribute to efforts of precise inter-continental frequency transfer.
It is very unlikely that time and frequency laboratories will deploy expensive and difficult to maintain radio telescopes which are necessary for VLBI operations. However, one could imagine a mutual benefit if a VLBI site is located in the vicinity of a timing laboratory and frequency is provided from this time lab over fiber to that VLBI site. This would imply that frequency standards at the VLBI sites can be omitted and the remotely provided frequency signals from the timing lab could be used for VLBI operations. One could then use global VLBI experiments to support inter-continental frequency transfer by a combination of GPS and VLBI on the observation level, as described in this study. However, the cable delay changes of the VLBI hardware and other time-dependent delays have to be monitored in order to gain benefit from adding VLBI. Estimation of such biases and delays, as performed in this study, helps to a certain extent, but monitoring and calibration of these inter-technique delays is crucial for the estimation of a common clock model among different space geodetic techniques. Efforts which could pave the way for this concept were reported in [28]. However such technology was neither available during CONT11 nor is it currently being used on an operational level. Monitoring and calibration of instrumental VLBI delays requires efforts to study all back-end components and their delay characteristics, a task which is expected to be more straightforward when phasing out analog systems and replacing them with digital equipment. In addition, one needs also to raise the awareness of such a demand in the geodetic VLBI user community in order to develop and deploy such delay calibration and monitoring systems.
Despite that VCE helps to weight observational data from the individual techniques, the number of VLBI observations still has to be increased in order to benefit even more when combining with GPS. Thus, the antenna and station design of the VLBI2010 concept [29], which will enable more and higher precise VLBI observations per hour, is expected to decrease the gap between GPS and VLBI in terms of temporal resolution. In addition, VLBI needs to observe continuously, which can only be achieved if unattended and automated station operation becomes reality.
Acknowledgments
The International GNSS Service [23] and the International VLBI Service for Geodesy and Astrometry [30] are acknowledged for providing the observational data. The design and the implementation of c5++ were huge efforts, requiring the participation and support of many individuals. We thank the anonymous reviewers for their valuable comments which helped to improve the paper.