ABSTRACT
We present the fractional distribution of spectroscopic subtypes, range and distribution of surface temperatures, and kinematical properties of the white dwarfs (WDs) within 25 pc of the Sun. There is no convincing evidence of halo WDs in the total 25 pc sample of 224 WDs. There is also little to suggest the presence of genuine thick disk subcomponent members within 25 pc. It appears that the entire 25 pc sample likely belongs to the thin disk. We also find no significant kinematic differences with respect to spectroscopic subtypes. The total DA to non-DA ratio of the 25 pc sample is 1.8, a manifestation of deepening envelope convection, which transforms DA stars with sufficiently thin H surface layers into non-DAs. We compare this ratio with the results of other studies. We find that at least 11% of the WDs within 25 pc of the Sun (the DAZ and DZ stars) have photospheric metals that likely originate from accretion of circumstellar material (debris disks) around them. If this interpretation is correct, then it suggests the possibility that a similar percentage have planets, asteroid-like bodies, or debris disks orbiting them. Our volume-limited sample reveals a pileup of DC WDs at the well-known cutoff in DQ WDs at Teff ∼ 6000 K. Mindful of small number statistics, we speculate on its possible evolutionary significance. We find that the incidence of magnetic WDs in the 25 pc sample is at least 8% in our volume-limited sample, dominated by cool WDs. We derive approximate formation rates of DB and DQ degenerates and present a preliminary test of the evolutionary scenario that all cooling DB stars become DQ WDs via helium convective dredge-up with the diffusion tail of carbon extending upward from their cores.
Export citation and abstract BibTeX RIS
1. INTRODUCTION
Extending the census of local white dwarfs (WDs) to increasingly large volumes of space around the Sun offers a plethora of crucial astrophysical insights, including (1) identification of the lowest luminosity, hence oldest, WDs in the immediate neighborhood of the Sun; (2) the distribution of WD spectroscopic subgroups and their mix of progenitor stellar populations; (3) a direct measurement of the local space density and mass density of WDs; and (4) a window into the history of star formation and stellar evolution in the Galactic plane as well as the age constraint of the Galactic disk (Liebert et al. 1988; Oswalt et al. 1996) by enabling the determination of the cool WD luminosity function. The sample of WDs out to 20 pc was presented by Holberg et al. (2008b) and Sion et al. (2009), who utilized available temperatures, gravities, and spectral types from the literature to attempt a full characterization of the local sample of WDs. With the publication of new atmospheric parameters and more accurate distances for a larger sample of local WDs out to 25 pc, the statistical and kinematical properties of the volume-limited sample of local WDs merit re-examination.
The local 25 pc WD sample is a well characterized, volume-limited population with a high degree of completeness. It contains a sample of 224 WDs including double degenerate systems. While it has an overall completeness of 65%, the 20 pc and 13 pc samples have completenesses of 85% and 100%, respectively. As such, it lends itself to a number of analyses that can be used to anchor larger, less complete spectroscopic and photometric surveys that are not volume-limited. The results of the current 25 pc sample will be discussed in J. Holberg et al. (2014, in preparation), which will include an evaluation of the spatial distribution, the space density, and the completeness of the sample, as well as the mass distribution, local luminosity function, and binary fraction.
In this paper, we discuss the kinematics and the distribution of WD temperature and spectroscopic subtypes in the 25 pc sample around the Sun, and propose tests of scenarios of cool WD spectral evolution. We briefly describe the enhanced 25 pc sample in Section 2. The kinematic properties of this sample are discussed in Section 3. The DQ and DC components of the 25 pc sample and their temperature distributions are discussed in Section 4. The magnetic and cool DZ stars are discussed in Sections 5 and 6, respectively. In Section 7 we describe preliminary tests of cool WD spectral evolution using the volume-limited 25 pc sample. Section 8 contains our conclusions.
2. CENSUS OF WHITE DWARFS OUT TO 25 pc
Table 1 presents the sample of WDs within 25 pc of the Sun. The basic observational data with which we examined the distribution of WD spectral types and the data used for space motions are given in Table 1, which contains by column: (1) the WD number, (2) the coordinates (R.A. and decl. are in decimal degrees), (3) Teff, (4) DIST (distance in parsecs), (5) PM (proper motion in arcsec yr−1), (6) PA (position angle in degrees), (7) the method of distance determination, denoted by p for trigonometric parallax and s for spectrophotometric distances, and (8) a reference abbreviation to the bibliography. Many of the distances are obtained from trigonometric parallaxes, the remainder from spectrophotometric distances.
Table 1. Observational Data Used in Space Motions
WD | Type | Teff | R.A. | Decl. | DIST | PM | PA | Method | Ref. |
---|---|---|---|---|---|---|---|---|---|
0000−345 | DCP8.1 | 6643 | 000.667 | −34.222 | 13.21 | 0.7578 | 169.046 | p | L20 |
0008+424 | DA6.8 | 7380 | 002.843 | +42.678 | 22.00 | 0.2328 | 191.648 | sp | L20 |
0009+501 | DAH7.6 | 6502 | 003.061 | +50.422 | 11.03 | 0.7150 | 219.920 | p | L20 |
0011−134 | DAH8.4 | 5992 | 003.553 | −13.183 | 19.49 | 0.8990 | 217.337 | p | L20 |
0011−721 | DA7.8 | 6325 | 003.457 | −71.831 | ⋅⋅⋅ | 0.3260 | 141.300 | sp | GBD |
0029−031 | DA11.3 | 4470 | 008.041 | −02.900 | 23.47 | 0.6505 | 76.305 | p | pi |
0038+555 | DQ4.6 | 10900 | 010.337 | +55.834 | 23.04 | 0.3396 | 103.627 | p | pi |
0038−226 | DQpec9.3 | 5529 | 010.358 | −22.350 | 9.04 | 0.6047 | 232.600 | p | L20 |
0046+051 | DZ7.4 | 6215 | 012.291 | +05.388 | 04.31 | 2.9780 | 155.538 | p | L20 |
0053−117 | DA7.1 | 13.959 | −11.458 | ⋅⋅⋅ | 0.4396 | 350.045 | sp | G11 | |
0108+048 | DA6.4 | 8530 | 015.958 | +05.075 | 21.32 | 0.3924 | 053.393 | p | G11 |
0108+277 | DA9.6 | 6428 | 017.686 | +27.970 | 13.8 | 0.2270 | 219.321 | p | L20 |
0115+159 | DQ5.6 | 9119 | 019.500 | +16.172 | 15.40 | 0.6480 | 181.805 | p | L20 |
0121−429 | DAH7.9 | 6299 | 021.016 | −42.677 | 18.31 | 0.5941 | 151.000 | p | L20 |
0123−262 | DC6.9 | 021.351 | −26.012 | ⋅⋅⋅ | 0.5945 | 154.8143 | sp | GBD | |
0123−460 | DA8.5 | 5898 | 021.325 | −45.752 | 24.90 | 0.7483 | 136.299 | sp | Sub08 |
0134+883 | DA2.8 | 18311 | 025.369 | +83.583 | 25.60 | 0.1500 | 306.870 | sp | G11 |
0135−052 | DA6.9 | 7118 | 024.497 | −04.995 | 12.34 | 0.6810 | 120.838 | p | L20 |
0141−675 | DA7.8 | 6248 | 025.754 | −67.308 | 09.72 | 1.0797 | 199.000 | p | L20 |
0145+360 | DA7.8 | 6470 | 27.168 | +36.258 | 24.2 | ⋅⋅⋅ | ⋅⋅⋅ | sp | Lim |
0148+467 | DA3.8 | 14005 | 028.012 | +47.001 | 15.85 | 0.1240 | 0.569 | p | L20 |
0148+641 | DA5.6 | 9016 | 027.963 | +64.431 | 17 | 0.2854 | 123.857 | sp | DR7 |
0208+396 | DAZ6.9 | 7264 | 032.836 | +39.922 | 16.72 | 1.1450 | 115.746 | p | L20 |
0210−508 | DQ+K1 | 6000 | 032.608 | −50.823 | 10.91 | 2.1920 | 72.666 | p | SL |
0213+396 | DA5.4 | 034.068 | 39.857 | ⋅⋅⋅ | 0.1873 | 239.876 | sp | G11 | |
0213+427 | DA9.0 | 5507 | 034.281 | +42.977 | 19.92 | 1.0470 | 125.065 | p | n L20 |
0227+050 | DA2.7 | 18779 | 037.569 | +05.264 | 24.30 | 0.0783 | 107.764 | p | G11 |
0230−144 | DA9.2 | 5477 | 038.157 | −14.197 | 15.62 | 0.6870 | 177.114 | p | L20 |
0231−054 | DA3.7 | 13550 | 038.532 | −05.194 | 22.7 | 0.2655 | 69.722 | sp | GBR11 |
0233−242 | DC9.3 | 5312 | 038.840 | −24.013 | 15.3 | 0.6220 | 189.015 | sp | L20 |
0236+259 | DA9.2 | 5500 | 039.832 | +26.165 | 21.00 | 0.3591 | 117.35 | p | Pi |
0243−026 | DAZ7.4 | 6839 | 041.628 | −02.456 | 21.23 | 0.5342 | 155.21 | p | G11 |
0245+541 | DAZ9.5 | 5319 | 042.151 | +54.389 | 10.35 | 0.5735 | 227.827 | p | L20 |
0252+497 | DA7.9 | 6370 | ⋅⋅⋅ | ⋅⋅⋅ | 16.7 | ⋅⋅⋅ | ⋅⋅⋅ | sp | Lim |
0255−705 | DAZ4.7 | 10560 | 044.071 | −70.369 | 21 | 0.6596 | 99.776 | sp | G11 |
0310−688 | DA3.3 | 16865 | 047.628 | −68.600 | 10.15 | 0.1112 | 158.097 | p | L20 |
0311−649 | DA4.0 | 11945 | 048.107 | −64.736 | 21.00 | 0.1661 | 101.107 | sp | Sub08 |
0322−019 | DAZ9.9 | 5195 | 051.296 | −01.820 | 16.80 | 0.9090 | 164.625 | p | L20 |
0326−273 | DA5.4 | 8483 | 052.203 | −27.317 | 17.36 | 0.8503 | 071.629 | p | L20 |
0340+198 | DA7.0 | 7160 | 055.846 | 19.970 | 18.4 | 0.178 | 24.55 | sp | Lim |
0341+182 | DQ7.7 | 6568 | 056.145 | +18.436 | 19.01 | 1.1990 | 159.771 | p | L20 |
0344+014 | DC9.9 | 5170 | 056.778 | +01.646 | ⋅⋅⋅ | 0.4730 | 150.400 | sp | L20 |
0357+081 | DA9.2 | 5478 | 060.111 | +08.235 | 17.82 | 0.5352 | 222.274 | p | L20 |
0413−077 | DA3.1 | 17100 | 063.839 | −07.656 | 05.04 | 4.0880 | 213.216 | p | L20 |
0416−594 | DA3.3 | 14000 | 064.122 | −59.302 | 18.23 | 0.1740 | 195.838 | p | SL |
0419−487 | DA8 | 6300 | 065.273 | −48.652 | 20.12 | 0.5402 | 178.302 | p | G11 |
0423+044 | DA | 5140 | 066.658 | +04.541 | 20.72 | 0.8469 | 131.936 | p | Pi |
0423+120 | DA8.2 | 6167 | 066.473 | +12.196 | 17.36 | 0.2446 | 335.866 | p | L20 |
0426+588 | DC7.1 | 7178 | 067.797 | +58.977 | 05.53 | 2.4267 | 147.602 | p | L20 |
0431−279 | DC9.5 | 5330 | 068.390 | −27.890 | 24.7 | 0.388 | 90.738 | p | Sub08 |
0431−360 | DA10.0 | 5153 | 068.232 | −35.395 | 25.0 | 0.301 | 84.1 | sp | Sub08 |
0433+270 | DA9.0 | 5629 | 069.187 | +27.164 | 17.85 | 0.2760 | 124.196 | p | L20 |
0435−088 | DQ8.0 | 6367 | 069.447 | −08.819 | 09.50 | 1.5740 | 171.103 | p | L20 |
0454+620 | DA4.3 | 11610 | 24.663 | +62.152 | 24.9 | 0.188 | 149.6 | sp | Lim |
0457−004 | DA4.7 | 10800 | 074.930 | −00.377 | 21.93 | 0.2926 | 142.778 | sp | L20 |
0503−174 | DAH9.5 | 5300 | 076.498 | −17.378 | 20.27 | 0.6876 | 14.4 | p | Pi |
0511+079 | DA7.7 | 6590 | 078.514 | +08.004 | 20.26 | 0.3642 | 216.178 | p | G11 |
0532+414 | DA6.8 | 7739 | 084.084 | +41.498 | 23.81 | 0.1668 | 284.903 | sp | G11 |
0548−001 | DQP8.3 | 6070 | 087.831 | −00.172 | 11.07 | 0.2510 | 025.810 | p | L20 |
0552−041 | DZ10.0 | 5182 | 088.789 | −04.168 | 06.41 | 2.3760 | 166.600 | p | |
0553+053 | DAP8.7 | 5785 | 089.106 | +05.363 | 07.99 | 1.0272 | 204.993 | p | L20 |
0615−591 | DB3.2 | 16714 | 094.063 | −59.206 | 23.80 | 0.379 | 166 | p | Pi |
0618+067 | DA8.1 | 5940 | 095.198 | +06.754 | 22.62 | 0.5352 | 91.392 | p | G11 |
0620−402 | DZ6 | 5919 | 095.423 | −40.217 | 21.50 | 0.379 | 166 | p | L20 |
0628−020 | DA | 6912 | 097.661 | −02.097 | 21.50 | 0.2088 | 253.300 | p | G11 |
0642−166 | DA2 | 25967 | 101.288 | −16.713 | 02.63 | 1.3394 | 204.057 | p | L20 |
0644+025 | DA6.8 | 22288 | 101.842 | +02.519 | 18.45 | 0.4234 | 272.572 | p | L20 |
0644+375 | DA2.4 | 22288 | 101.908 | +37.515 | 15.40 | 0.9624 | 193.561 | p | L20 |
0649+639 | DA8.1 | 6230 | 103.560 | +63.932 | 21.1 | 0.200 | 225.0 | sp | Lim |
0651−398A | DA7.0 | 7222 | 103.397 | −39.925 | 25.10 | 0.2125 | 341.906 | sp | Sub08 |
0655−390 | DA7.9 | 6311 | 104.274 | −39.159 | 17.2 | 0.3400 | 242.600 | sp | GBD |
0657+320 | DA10.1 | 4888 | 105.215 | +31.962 | 18.69 | 0.6910 | 149.362 | p | L20 |
0659−063 | DA7.7 | 6627 | 105.478 | −06.463 | 12.34 | 0.8984 | 184.981 | p | L20 |
0706+377 | DQ7.6 | 6590 | 107.559 | +37.672 | 24.27 | 0.3577 | 220.010 | p | Pi |
0708−670 | DC9.9 | 5097 | 107.217 | −67.108 | 17.5 | 0.2460 | 246.300 | sp | GBD |
0727+482.1 | DA10.0 | 4934 | 112.678 | +48.199 | 11.11 | 1.2868 | 190.070 | p | L20 |
0727+482.2 | DA10.1 | 4926 | 112.697 | +48.173 | 11.11 | 1.2868 | 190.070 | p | L20 |
0728+642 | DAP11.1 | 5135 | 113.378 | +64.157 | 13.4 | 0.2660 | 171.352 | sp | L20 |
0736+053 | DQZ6.5 | 7871 | 114.827 | +05.227 | 03.50 | 1.2590 | 214.574 | p | L20 |
0738−172 | DZA6.6 | 7650 | 115.086 | −17.413 | 09.09 | 1.2634 | 116.600 | p | L20 |
0743−336 | DC10.6 | 4462 | 116.410 | −33.931 | 15.19 | 1.7360 | 352.670 | p | L20 |
0744+112 | DA6.2 | 8160 | 116.875 | 11.126 | 25.7 | 0.1907 | 161.66 | sp | Lim |
0747+073.1 | DC10.4 | 4366 | 117.563 | +07.193 | 18.28 | 1.8049 | 173.414 | p | L20 |
0747+073.2 | DC12.0 | 4782 | 117.560 | +07.196 | 18.28 | 1.8049 | 173.414 | p | L20 |
0749+426 | DC11.7 | 4585 | 118.305 | +42.500 | 17.8 | 0.420 | 165.845 | sp | L20 |
0751−252 | DA9.8 | 5085 | 118.485 | −25.400 | 17.68 | 0.3622 | 304.700 | p | L20 |
0752−676 | DA8.8 | 5735 | 118.284 | −67.792 | 07.89 | 2.1499 | 135.867 | p | L20 |
0753+417 | DA7.3 | 6880 | 119.129 | +41.664 | 24 | 0.3481 | 181.316 | sp | Kilic-10 |
0805+356 | DA7.3 | 6900 | 122.296 | +35.465 | 21.1 | 0.1051 | 220.873 | sp | Trem11 |
0806−661 | DQ4.9 | 10205 | 121.723 | −66.304 | 19.16 | 0.4468 | 130.400 | p | L20 |
0810+489 | DC6.9 | 7300 | 123.546 | +48.758 | 17.00 | 0.258 | 166.5 | p | GBD |
0816−310 | DZ7.6 | 6463 | 124.667 | −31.172 | 23.80 | 0.8163 | 164.074 | sp | GBD |
0821−669 | DA9.8 | 5088 | 125.361 | −67.055 | 10.65 | 0.7623 | 329.500 | p | L20 |
0827+328 | DA6.9 | 7490 | 127.664 | +32.696 | 22.27 | 0.5412 | 196.090 | p | L20 |
0839−327 | DA5.5 | 9081 | 130.384 | −32.942 | 08.81 | 1.7020 | 322.700 | p | G11 |
0840−136 | DZ10.3 | 4874 | 130.701 | −13.786 | 19.3 | 0.2720 | 263.000 | sp | L20 |
0843+358 | DZ6 | 9041 | 131.685 | +35.642 | 23.1 | 0.174 | 244.466 | sp | GBD |
0856+331 | DQ5.1 | 9920 | 134.811 | +32.953 | 20.49 | 0.334 | 269.657 | p | Pi |
0912+536 | DCP7 | 7235 | 138.983 | +53.423 | 10.30 | 1.5630 | 223.997 | p | L20 |
0946+534 | DQ6.2 | 8100 | 147.571 | +53.254 | 22.98 | 0.2642 | 259.753 | p | Pi |
0955+247 | DA5.8 | 8621 | 149.451 | +24.548 | 24.44 | 0.4200 | 219.848 | p | L20 |
0959+149 | DC7 | 7200 | 150.455 | +14.689 | 22.2 | 0.339 | 269.493 | p | GJ |
1008+290 | DQpec11.0 | 152.923 | 28.766 | ⋅⋅⋅ | 0.7201 | 189.593 | p | Pi | |
1009−184 | DZ8.5 | 6036 | 153.007 | −18.725 | 18.30 | 0.5114 | 269.000 | p | L20 |
1012+083.1 | DA7.5 | 6750 | 153.760 | +08.109 | 25.80 | 0.3339 | 300.405 | sp | G11 |
1019+637 | DA7.2 | 6742 | 155.787 | +63.461 | 16.33 | 0.3790 | 053.160 | p | L20 |
1033+714 | DC10.3 | 4727 | 159.260 | +71.183 | 15.15 | 1.9170 | 256.008 | p | L20 |
1036−204 | DQpecP10.2 | 4694 | 159.731 | −20.682 | 14.28 | 0.6100 | 334.000 | p | L20 |
1043−188 | DQ8.1 | 5780 | 161.412 | −19.114 | 12.15 | 1.9780 | 251.636 | p | L20 |
1055−072 | DA6.8 | 7491 | 164.396 | −07.523 | 13.42 | 0.8270 | 276.328 | p | L20 |
1105−048 | DA3.5 | 15141 | 166.999 | −05.157 | 24.2 | 0.4445 | 188.148 | sp | G11 |
1116−470 | DC8.6 | 5801 | 169.613 | −47.365 | 17.9 | 0.3220 | 275.100 | sp | GBD |
1121+216 | DA6.7 | 7434 | 171.054 | +21.359 | 13.42 | 1.0400 | 269.240 | p | L20 |
1124+595 | DA4.8 | 10747 | 171.718 | +59.321 | 25 | 0.1569 | 108.204 | sp | L20 |
1132−325 | DC | 173.623 | −32.832 | 09.53 | 0.9400 | 038.955 | p | L20 | |
1134+300 | DA2.4 | 22469 | 174.271 | +29.799 | 15.31 | 0.1480 | 267.948 | p | L20 |
1142−645 | DQ6.4 | 7966 | 176.458 | −64.841 | 04.62 | 2.6876 | 097.500 | p | L20 |
1148+687 | DA7.6 | ⋅⋅⋅ | 177.718 | +68.521 | 16.8 | ⋅⋅⋅ | ⋅⋅⋅ | sp | DR7 |
1149−272 | DQ8.1 | 6200 | 177.900 | −27.539 | 24.40 | 0.229 | 283.126 | sp | Sub08 |
1202−232 | DAZ5.8 | 8767 | 181.361 | −23.553 | 10.82 | 0.2458 | 16.600 | p | L20 |
1208+576 | DAZ8.6 | 6200 | 182.872 | +57.404 | 20.44 | 0.5486 | 132.414 | p | G11 |
1214+032 | DA8.0 | 6272 | 184.216 | +02.968 | 19.72 | 0.6947 | 291.357 | sp | Pi |
1223−659 | DA6.6 | 7594 | 186.625 | −66.205 | 16.25 | 0.1858 | 186.900 | p | G11 |
1236−495 | DA4.3 | 11599 | 189.708 | −49.800 | 16.39 | 0.4902 | 255.709 | p | L20 |
1241−798 | DC/DQ | 9556 | 191.219 | −80.157 | 22.10 | 0.5524 | 309.858 | sp | Sub08 |
1242−105 | DA6.3 | 191.219 | −10.852 | ⋅⋅⋅ | 0.3488 | 257.079 | sp | GBD | |
1257+037 | DA9.0 | 5616 | 195.037 | +03.478 | 16.58 | 0.9696 | 206.195 | p | L20 |
1309+853 | DAP9 | 5440 | 197.171 | +85.041 | 16.47 | 0.3213 | 140.811 | p | L20 |
1310+583 | DA4.8 | 10544 | 198.241 | +58.086 | 21.1 | 0.1995 | 112.708 | sp | G11 |
1310−472 | DC11.9 | 4158 | 198.248 | −47.468 | 15.03 | 2.2047 | 105.253 | p | L20 |
1315−781 | DC8.8 | 5619 | 199.856 | −78.391 | 19.17 | 0.4700 | 139.5 | p | Pi |
1327−083 | DA3.6 | 14571 | 202.556 | −08.574 | 16.86 | 1.2049 | 246.761 | p | L20 |
1334+039 | DA11 | 4971 | 204.132 | +03.679 | 08.23 | 3.8800 | 252.775 | p | L20 |
1344+106 | DAH7.1 | 7059 | 206.851 | +10.360 | 20.04 | 0.9032 | 260.569 | p | L20 |
1337+705 | DAZ2.5 | 20464 | 204.710 | +70.285 | 24.79 | 0.405 | 266.035 | p | G11 |
1338+052 | DC11.6 | 205.340 | 5.012 | ⋅⋅⋅ | 0.438 | 271.6 | sp | Say | |
1339−340 | DA9.5 | 5361 | 206.508 | +57.009 | 21.20 | 0.2758 | 315.881 | sp | GBD |
1344+572 | DA3.8 | 13389 | 206.510 | +57.008 | 20 | 0.273 | 315.9 | sp | G11 |
1345+238 | DA11 | 4581 | 207.012 | +23.579 | 12.06 | 1.4960 | 274.637 | p | L20 |
1350−090 | DAP5 | 9518 | 208.314 | −09.275 | 22.73 | 0.3618 | 174.226 | sp | G11 |
1401+457 | DC19 | 2600 | 210.853 | +45.558 | 24.00 | 0.2840 | 251.947 | sp | Kilic10 |
1425−811 | DAV4.2 | 12098 | 218.282 | −80.157 | 22.72 | 0.5524 | 201.418 | p | G11 |
1436−781 | DA8.1 | 6270 | 220.714 | −78.398 | 24.65 | 0.4096 | 275.1 | p | Pi |
1444−174 | DC10.2 | 4982 | 221.855 | −17.704 | 14.49 | 1.1440 | 252.643 | p | L20 |
1532+129 | DZ6.7 | 7500 | 233.774 | +12.795 | 22 | 0.2464 | 222.039 | sp | Koe11 |
1538+333 | DA5.6 | 8940 | 235.139 | +33.147 | 22.72 | 0.186 | 296.152 | p | Pi |
1542−275 | DB4.0 | 237.658 | 27.656 | ⋅⋅⋅ | 0.246 | 235.9 | sp | Ber11 | |
1544−377 | DA4.8 | 10610 | 236.875 | −37.918 | 15.24 | 0.4685 | 242.838 | p | L20 |
1609+135 | DA5.4 | 9041 | 242.856 | +13.371 | 18.34 | 0.5510 | 178.513 | p | L20 |
1620−391 | DA2.1 | 25985 | 245.890 | −39.229 | 12.86 | 0.0755 | 89.962 | p | L20 |
1625+093 | DA7.3 | 7038 | 246.972 | +09.204 | 23.36 | 0.4872 | 192.325 | p | G11 |
1626+368 | DZA6.0 | 8507 | 247.104 | +36.771 | 15.94 | 0.8881 | 326.668 | p | L20 |
1630+089 | DA9.0 | 5640 | 177.717 | +68.521 | 13.2 | 0.4 | ⋅⋅⋅ | sp | Lim |
1632+177 | DAZ5.0 | 10225 | 248.674 | +17.609 | ⋅⋅⋅ | 0.0885 | 108.435 | sp | L20 |
1633+433 | DAZ7.7 | 6608 | 248.755 | +43.293 | 15.10 | 0.3730 | 144.151 | p | L20 |
1633+572 | DQ8.2 | 5958 | 248.589 | +57.169 | 14.45 | 1.6440 | 317.229 | p | L20 |
1639+537 | DAH6.7 | 7510 | 250.238 | +53.685 | 21.09 | 0.2369 | 212.416 | p | Pi |
1647+591 | DAV4.1 | 12738 | 252.106 | +59.056 | 10.95 | 0.3236 | 154.498 | p | L20 |
1655+215 | DAB5.4 | 9179 | 254.291 | +21.446 | 23.25 | 0.5820 | 178.040 | p | L20 |
1658+440 | DAP1.7 | 30510 | 254.951 | +44.017 | 22.00 | 0.1012 | 341.869 | sp | G11 |
1705+030 | DZ7.7 | 6584 | 257.033 | +02.960 | 17.54 | 0.3790 | 180.907 | p | L20 |
1748+708 | DQ9.0 | 5570 | 267.033 | +70.876 | 06.07 | 1.6810 | 311.394 | p | L20 |
1756+143 | DA9.0 | 5466 | 269.595 | +14.293 | 22.40 | 1.0054 | 235.045 | sp | GBD |
1756+827 | DA6.9 | 7214 | 267.458 | +82.773 | 15.64 | 3.5897 | 336.542 | p | L20 |
1814+134 | DA9.5 | 5251 | 274.277 | +13.473 | 14.22 | 1.2070 | 201.500 | p | L20 |
1817−598 | DA5.8 | 4960 | 275.497 | −59.863 | 24.87 | 0.3653 | 194.911 | p | Pi |
1820+609 | DA10.5 | 4919 | 275.332 | +61.018 | 12.78 | 0.7133 | 168.517 | p | L20 |
1829+547 | DQP8.0 | 6345 | 277.584 | +54.790 | 14.97 | 0.3991 | 317.234 | p | L20 |
1840+042 | DA5.8 | 9090 | 280.857 | +04.339 | 24.87 | 0.187 | 296.935 | p | G11 |
1900+705 | DAP4.2 | 11835 | 285.042 | +70.664 | 12.98 | 0.5064 | 10.467 | p | L20 |
1911+536 | DA2.9 | 17670 | 288.202 | +53.720 | 22.1 | 0.018 | 225.0 | sp | Lim |
1912+143 | DA7.3 | 6940 | 288.650 | +14.473 | 19.4 | 0.161 | 225.0 | sp | Lim |
1917+386 | DC7.9 | 6459 | 289.744 | +38.722 | 11.69 | 0.2510 | 174.028 | p | L20 |
1917−077 | DBQZ4.9 | 10396 | 290.145 | −07.666 | 10.08 | 0.1740 | 200.602 | p | L20 |
1919+145 | DA3.3 | 15280 | 290.418 | +14.678 | 19.80 | 0.0743 | 203.806 | p | L20 |
1935+276 | DA4.2 | 12130 | 294.307 | +27.721 | 17.95 | 0.4361 | 088.686 | p | L20 |
1953−011 | DC6.4 | 7920 | 299.121 | −01.042 | 11.38 | 0.8270 | 212.314 | p | L20 |
2002−110 | DA10.5 | 4800 | 301.395 | −10.948 | 17.33 | 1.0740 | 095.523 | p | L20 |
2007−303 | DA3.5 | 14454 | 302.736 | −30.218 | 17.09 | 0.4280 | 233.492 | p | L20 |
2008−600 | DC9.9 | 5080 | 303.132 | −59.947 | 16.55 | 1.4276 | 166.100 | p | L20 |
2008−799 | DA8.5 | 5800 | 304.207 | −79.764 | 24.96 | 0.4339 | 127.979 | p | Pi |
2011+065 | DQ7 | 6400 | 303.481 | +06.712 | 22.37 | 0.6297 | 203.39 | p | Pi |
2032+248 | DA2.4 | 19983 | 308.591 | +25.063 | 14.65 | 0.6920 | 215.554 | p | L20 |
2039−202 | DA2.5 | 19207 | 310.644 | −20.076 | 21.10 | 0.3672 | 104.831 | p | G11 |
2039−682 | DA3.1 | 15855 | 311.089 | −68.089 | 22.00 | 0.3269 | 144.462 | sp | G11 |
2040−392 | DA4.5 | 10830 | 310.955 | −39.055 | 22.63 | 0.306 | 179 | p | G11 |
2047+372 | DA3.6 | 14070 | 312.277 | +37.470 | 17.28 | 0.2190 | 047.150 | p | L20 |
2048+263 | DA9.7 | 5200 | 312.586 | +26.511 | 20.08 | 0.5149 | 235.044 | p | L20 |
2048−250 | DA6.6 | 7630 | 312.749 | −24.867 | 22 | 0.2776 | 129.885 | sp | GBD |
2054−050 | DC10.9 | 4620 | 314.199 | −04.844 | 17.05 | 0.8020 | 106.562 | p | L20 |
2058+342 | DB4.1 | 315.089 | 34.439 | ⋅⋅⋅ | 0.168 | 42.6 | sp | Ber11 | |
2105−820 | DA4.7 | 10620 | 318.320 | −81.820 | 17.06 | 0.5164 | 146.372 | p | L20 |
2111+072 | DA7.8 | 6470 | 317.370 | +07.451 | 24.1 | 0.341 | 70.289 | sp | Lim |
2115−560 | DA6 | 9736 | 319.902 | −55.837 | 22.00 | 0.4652 | 115.463 | sp | G11 |
2117+539 | DA3.6 | 13990 | 319.734 | +54.211 | 19.72 | 0.2130 | 336.371 | p | L20 |
2118−388 | DC9.6 | 5244 | 320.523 | −38.643 | 22.00 | 0.1786 | 112.009 | sp | Sub08 |
2119+040 | DA9.0 | 320.551 | 4.232 | ⋅⋅⋅ | 0.3897 | 28.341 | sp | Say | |
2126+734 | DA3.8 | 16,104 | 321.740 | 73.645 | 21.23 | 0.2915 | 171.119 | p | G11 |
2133−135 | DA5.0 | 9736 | 324.068 | −13.309 | 20.40 | 0.2935 | 118.486 | sp | Sub08 |
2138−332 | DZ7 | 7240 | 325.489 | −33.008 | 15.62 | 0.2100 | 228.500 | p | L20 |
2140+207 | DQ6.1 | 8200 | 325.670 | +20.999 | 12.51 | 0.6819 | 199.445 | p | L20 |
2149+021 | DA2.8 | 17353 | 328.105 | +02.388 | 24.50 | 0.3003 | 177.328 | p | G11 |
2151−015 | DA6 | 8400 | 328.526 | −01.285 | 19.60 | 0.2851 | 178.191 | sp | G11 |
2154−512 | DQ8.3 | 6100 | 329.421 | −51.006 | 16.12 | 0.3746 | 184.738 | p | L20 |
2159−754 | DA5.6 | 9040 | 331.086 | −75.223 | 15.62 | 0.2042 | 238.300 | p | L20 |
2210+565 | DA3.0 | 16790 | 332.973 | +56.829 | 18.1 | 0.147 | 217.0 | sp | Say |
2211−392 | DA8.1 | 6920 | 333.638 | −38.983 | 18.69 | 1.068 | 109.6 | p | Say |
2211−392 | DA8.1 | 6920 | 333.644 | −38.985 | 18.79 | 1.0560 | 110.100 | p | Say |
2215+386 | DC10.6 | 4700 | 334.448 | +37.130 | 25.12 | 0.4691 | 78.69 | p | Pi |
2226−754 | DC11.9 | 4230 | 337.665 | −75.232 | 12.8 | 1.8680 | 167.500 | sp | L20 |
2226−755 | DC12.1 | 4177 | 337.638 | −75.255 | 14.0 | 1.8680 | 167.500 | sp | L20 |
2246+223 | DA4.7 | 10647 | 342.273 | +22.608 | 19.04 | 0.5253 | 83.551 | p | L20 |
2248+293 | DA9 | 5580 | 342.845 | +29.662 | 20.92 | 1.2575 | 83.745 | p | G11 |
2251−070 | DZ12.6 | 4000 | 343.472 | −06.781 | 08.51 | 2.5718 | 105.600 | p | L20 |
2253+054 | DA9 | 5600 | 343.982 | +05.755 | 24.46 | 0.4471 | 127.101 | p | Pi |
2311−068 | DQ6.8 | 7440 | 348.604 | −06.546 | 25.1 | 0.3815 | 244.039 | p | Pi |
2322+137 | DA10.7 | 4700 | 351.332 | +14.060 | 22.27 | 0.37 | 71.565 | p | L20 |
2326+049 | DAV4.3 | 12206 | 352.198 | +05.248 | 13.62 | 0.4934 | 236.406 | p | G11 |
2336−079 | DA4.6 | 10938 | 354.711 | −07.688 | 15.94 | 0.0500 | 140.000 | p | L20 |
2341+322 | DA4.0 | 13128 | 355.961 | +32.546 | 17.60 | 0.2290 | 252.150 | p | L20 |
2347+292 | DA9 | 5810 | 357.479 | +29.567 | 22.39 | 0.5065 | 185.666 | p | Pi |
2351−335 | DA5.7 | 8850 | 358.504 | −33.275 | 23.35 | 0.5081 | 219.4 | p | G11 |
2359−434 | DA5.9 | 8648 | 000.544 | −43.165 | 08.16 | 0.8878 | 138.400 | p | L20 |
Notes. s, spectrophotometric; p, trigonometric parallax; a, weighted mean average. References. G11: Gianninas et al. (2011); Lim: Limoges et al. (2013); Say: Sayres et al. (2012); Pi: Trig. Parallax; DR7: J. Holberg et al. (2014, in preparation); Sub08: Subasavage et al. (2008); GBR11: Gianninas et al. (2011); Sub07: Subasavage et al. (2007); Ber11: Bergeron et al. (2011); GJ: Gliese & Jarheise (1995); Kilic10: Kilic et al. (2010); Koe11: Koester et al. (2011); Trem11: Tremblay et al. (2011).
A substantial fraction of the data in Table 1 was taken from the detailed survey of the local WDs out to 20 pc by Holberg et al. (2008b) and Sion et al. (2009). To this sample of 129 WDs, we have added 95 WDs out to a volume of 25 pc radius, giving a total sample of 224 WDs including double degenerates known out to 25 pc. As in Holberg et al. (2008b) and Sion et al. (2009), the photometric distances were computed based on spectroscopic and photometric measurements following the methods described in Holberg et al. (2008a). Proper Motions are taken from the McCook and Sion Catalog, or where available, were determined from NOMAD. Radial velocities were available from the literature for approximately 50% of our sample, and correspond either to direct measurements of the WD, or the system velocities or radial velocities of the main sequence companions (McCook & Sion 1999 and references therein; Maxted et al. 2000; Silvestri et al. 2001, 2002; Pauli et al. 2003, 2006). For radial velocities derived from individual WDs, we applied corrections for the gravitational redshift based on the individual masses and radii of each star. These mass and radius determinations were interpolated from within the synthetic photometric tables described in Holberg & Bergeron (2006) and were based on temperatures and gravities given in Holberg et al. (2008b, hereafter, LS08). Using the spectral types and temperatures given in LS08 and Holberg (2008), we assembled the local population of WDs lying within 25 pc of the Sun using a number of compilations, including Gliese & Jarheise (1995), LS08, Sion et al. (2009), Sayres et al. (2012), Bergeron et al. (2011), Giammichele et al. (2012), Dufour et al. (2007), Limoges et al. (2013), Subasavage et al. (2007, 2008), Gianninas et al. (2011), Kilic et al. (2010), Koester et al. (2011), and Tremblay et al. (2011).
We summarize the percentage breakdown of spectral subtypes among the 25 pc sample of local WDs in Table 2. As expected, the DA stars dominate the sample. If we take the total DA sample to include DAZ stars and magnetic DA stars, then there are 150 DA stars within 25 pc.
Table 2. Distribution of WD Spectral Subtypes within 25 pc
Spectral Type | Range of Teff | Number of Stars | % of Total |
---|---|---|---|
DA | 4590–25193 | 125 | 54% |
DAZ | 5093–20464 | 11 | 5% |
DAH/DAP | 4500–30510 | 14 | 6% |
Magnetic Non-DA | 5 | ||
DB | 16714 | 2 | 0.8% |
DBQZ | 10200 | 1 | 0.4% |
DC | 2600–7300 | 28 | 12% |
DCP | 6010 | 3 | 1.3% |
DQ | 5590–10900 | 23 | 8% |
DQP | 4948–6070 | 3 | 0.9% |
DQZ | 7740 | 1 | 0.4% |
DZ | 4000–7500 | 13 | 5% |
DZA | 7600–8440 | 2 | 0.9% |
Download table as: ASCIITypeset image
In Figure 1 we display a histogram with the number versus Teff distribution function of DA stars in the lower panel and non-DA stars in the upper panel. The skewing of their distribution toward lower temperatures not only reflects the predominance of cool DAs and cool non-DAs in a sample so close to the Sun, but the variation of this ratio, DA/non-DA, as a function of Teff, also holds key physical significance to our understanding of WD spectral evolution.
Figure 1. Histogram of the number vs. surface temperature distribution function for all DA white dwarfs (lower panel) and all non-DA white dwarfs (upper panel) within 25 pc of the Sun.
Download figure:
Standard image High-resolution imageThe overall DA to non-DA ratio of the 25 pc sample is 1.83. This is a slightly larger ratio than found in the 20 pc sample and is likely a lower limit since a few of the cool non-DAs (especially DC stars) may actually prove to be H-dominated. Sion (1984) uncovered the first empirical evidence of this transformation of WD surface compositions and attributed the lowering of the DA/non-DA ratio with decreasing Teff to the mixing and dilution of hydrogen into the deepening helium convection zone (Sion 1984; see also Greenstein 1986). Sion (1984) estimated that this convective mixing away of surface hydrogen in cooling DA stars occurs at Teff ∼ 10–12,000 K. Subsequent more detailed work with evolutionary models (Tremblay & Bergeron 2008; Bergeron et al. 2001), using a larger, more homogenous sample of WDs, presented a more complex picture of spectral evolution at temperatures below 15,000 K. They showed that as a DA WD cools, the bottom of the hydrogen convection zone eventually reaches the helium convection zone but only if the hydrogen layer is thin enough, with the actual temperature at which the conversion occurs depending on the thickness of the hydrogen layer. In other words, DA WDs with thicker hydrogen layers will mix at lower Teff. They showed that the H layer mass that would mix at a given temperature depends on the assumed convective efficiency, with ∼85% of all DA stars between 10,000 K and 15,000 K having hydrogen layer masses large enough for them to remain recognized as DA stars down to 8000 K. It is clear from the results of Tremblay & Bergeron (2008) that the details of WD spectral evolution remain murky below 12,000 K. This can only be clarified with further theoretical modeling predictions confronted by ever larger samples of cool WDs with high quality spectra.
3. KINEMATICS OF THE LOCAL WHITE DWARFS
The vector components of the space motions are U, V, and W, where U is positive in the direction of the galactic anti-center, V is positive in the direction of the galactic rotation, and W is positive in the direction of the north Galactic pole (Wooley et al. 1970). The U, V, and W components and total motions were computed along with the average velocities and velocity dispersions for the different spectroscopic subtypes in the 25 pc sample in Table 1. In Table 3, we present the computed vector components of the space motion for each WD in Table 1. We note that for some of the WDs in Table 1, there are two sets of space motions from slightly different input parameters, such as proper motion and position angles. For these objects, two different sets of U, V, W, T values were computed and used to compute the averages and dispersions tabulated in Table 4. These mean velocities and velocity dispersions reveal no clear evidence that different spectral subtypes have significantly different space motions.
Table 3. Space Motions of White Dwarfs within 25 pc
WD No. | Type | U | V | W | T |
---|---|---|---|---|---|
0000−345 | DCP9 | −11.9 | −43.7 | 3.2 | 45.4 |
0008+424 | DA6.8 | −10.0 | −1.9 | −17.4 | 20.2 |
0009+501 | DAH7.7 | −28.1 | 8.6 | −24.1 | 38.0 |
0011−134 | DCH8.4 | −75.5 | −31.1 | −9.8 | 82.3 |
0011−721 | DA8.0 | 10.3 | −23.2 | 12.2 | 28.2 |
0029−031 | DA11.3 | 68.3 | −23.0 | 2.6 | 72.1 |
0038+555 | DQ4.6 | 39.4 | −2.0 | −11.9 | 41.2 |
0038−226 | DQ9.3 | −24.9 | −4.6 | −1.2 | 25.3 |
0046+051 | DZ8.1 | −2.8 | −53.6 | −30.3 | 61.6 |
0108+048 | DA6.4 | 38.2 | −0.1 | 11.8 | 40.0 |
0108+277 | DAZ9.6 | −11.9 | 0.3 | −9.9 | 15.5 |
0115+159 | DQ6 | −21.4 | −27.2 | −32.0 | 47.2 |
0121−429 | DAH7.9 | −0.7 | −46.8 | 11.1 | 48.1 |
0123−460 | DA8.5 | 24.3 | −91.0 | 24.9 | 97.4 |
0134+883 | DA2.8 | −11.1 | 5.1 | 6.1 | 13.7 |
0135−052 | DA6.9 | 16.8 | −37.2 | −1.6 | 40.9 |
0141−675 | DA7.8 | −21.8 | −22.8 | 21.5 | 38.2 |
0148+467 | DA3.8 | 2.6 | 1.8 | 8.3 | 8.8 |
0148+641 | DA5.6 | 12.3 | −11.2 | −6.8 | 18.0 |
0208+396 | DAZ7.0 | 43.0 | −62.9 | −6.7 | 76.5 |
0210−510 | DA10 | 85.6 | −42.1 | 24.0 | 98.4 |
0213+427 | DA9.4 | 37.8 | −67.3 | −19.8 | 79.8 |
0227+050 | DA2.7 | −0.1 | −8.9 | 7.1 | 11.4 |
0230−144 | DC9.5 | −20.7 | −43.3 | −13.3 | 49.9 |
0231−054 | DA3.7 | 68.5 | −6.7 | −56.4 | 89.0 |
0233−242 | DC9.3 | −24.2 | −34.1 | −9.8 | 43.0 |
0236+259 | DA9.2 | 29.1 | −21.1 | −8.9 | 37.0 |
0243−026 | DAZ7.4 | 8.9 | −51.6 | −34.5 | 62.7 |
0245+541 | DAZ9.7 | −16.4 | 10.9 | −24.2 | 31.3 |
0255−705 | DAZ4.7 | 22.2 | −82.0 | −0.6 | 84.9 |
0310−688 | DA3.1 | 0.1 | −4.2 | 2.8 | 5.1 |
0311−649 | DA4.0 | 7.9 | −12.8 | 9.5 | 17.8 |
0322−019 | DAZ9.7 | −21.5 | −66.8 | −20.7 | 73.2 |
0326−273 | DA5.4 | 46.7 | −29.7 | 47.2 | 72.8 |
0341+182 | DQ7.7 | −16.0 | −94.9 | −23.8 | 99.1 |
0344+014 | DQ9.9 | −7.9 | −43.8 | −4.9 | 44.8 |
0357+081 | DC9.2 | −25.3 | −4.3 | −36.8 | 44.9 |
0413−077 | DAP3.1 | −44.2 | −34.8 | −73.0 | 92.2 |
0419−487 | DA8 | −1.2 | −91.1 | −57.4 | 107.7 |
0416−594 | DA3.8 | −4.9 | −5.7 | −1.6 | 7.7 |
0423+044 | DA9 | −6.9 | −80.5 | 17.2 | 82.6 |
0423+120 | DA8.2 | 5.3 | 18.1 | 3.8 | 19.3 |
0426+588 | DC7.1 | 0.9 | −35.4 | −3.7 | 35.6 |
0431−360 | DA10.0 | 10.8 | −19.6 | 27.0 | 35.1 |
0431−279 | DC9.5 | 11.5 | −28.8 | 33.3 | 45.5 |
0433+270 | DA9.3 | −0.1 | −19.8 | 7.0 | 21.1 |
0435−088 | DQ8.0 | −25.1 | −63.2 | −21.6 | 71.4 |
0457−004 | DA4.7 | −6.1 | −23.8 | 2.5 | 24.7 |
0503−174 | DAH9.5 | 32.2 | 42.5 | 41.0 | 67.2 |
0511+079 | DA7.7 | −14.5 | −9.2 | −30.6 | 35.1 |
0532+414 | DA6.8 | 1.2 | 12.4 | −13.4 | 18.2 |
0548−001 | DQP8.3 | 4.6 | 6.3 | 10.5 | 13.1 |
0552−041 | DZ11.8 | −25.3 | −65.7 | −20.2 | 73.3 |
0553+053 | DAP8.9 | −12.2 | −19.8 | −31.2 | 38.9 |
0628−020 | DA | −5.2 | −7.7 | −17.7 | 20.0 |
0615−591 | DB3.2 | −11.3 | −0.1 | 7.1 | 13.3 |
0618+067 | DA8.1 | −9.0 | −26.9 | 49.9 | 57.4 |
0620−402 | DZ6 | −13.9 | −25.0 | −7.2 | 29.5 |
0627+299 | DA5 | −0.80 | −1.4 | −0.6 | 1.7 |
0628−020 | DA | 61.0 | −34.5 | −27.2 | 75.2 |
0642−166 | DA2 | −0.6 | −10.2 | −14.5 | 17.7 |
0644+025 | DA8 | 9.4 | 15.1 | −30.9 | 35.7 |
0644+375 | DA2.5 | −19.7 | −39.6 | −32.7 | 55.1 |
0651−398A | DA7.0 | 5.2 | 28.7 | 8.9 | 30.5 |
0655−390 | DA8.0 | 2.7 | 2.3 | −28.2 | 28.4 |
0657+320 | DC10.1 | −31.7 | −52.9 | 13.2 | 63.1 |
0659−063 | DA7.8 | −19.7 | −37.5 | −29.5 | 51.6 |
0708−670 | DC | 4.7 | 4.9 | −19.9 | 21.1 |
0706+377 | DQ7.6 | −7.2 | −13.0 | −31.6 | 34.9 |
0727+482.1 | DA10.0 | −14.6 | −40.5 | −14.7 | 45.5 |
0727+482.2 | DA10.0 | −14.9 | −41.1 | −14.9 | 46.3 |
0728+642 | DAP11.2 | −4.5 | −9.2 | 3.9 | 11.0 |
0736+053 | DQZ6.5 | 0.6 | −12.0 | −18.4 | 21.9 |
0738−172 | DAZ6.7 | −30.5 | −29.2 | 30.2 | 51.9 |
0743−336 | DC10.6 | 49.4 | 67.6 | 61.4 | 103.9 |
0747+073.1 | DA12.1 | −76.7 | −126.3 | −49.0 | 155.7 |
0747+073.2 | DA11.9 | −76.7 | −126.3 | −49.0 | 155.7 |
0749+426 | DC11.7 | −17.8 | −29.6 | 5.6 | 35.0 |
0751−252 | DA10.0 | 19.1 | 15.5 | −13.8 | 28.3 |
0752−676 | DC10.3 | −29.3 | −15.4 | 13.6 | 35.9 |
0753+417 | DA7.3 | −13.8 | −28.6 | −3.5 | 31.9 |
0806−661 | DQ4.2 | −17.2 | −6.5 | 7.3 | 19.8 |
0805+356 | DA7.3 | 0.5 | −4.9 | −6.6 | 8.3 |
0810+489 | DC6.9 | −9.0 | −15.1 | 5.0 | 18.3 |
0810+489 | DC6.9 | −14.5 | −22.8 | 8.6 | 28.3 |
0816−310 | DZ7.6 | −45.0 | −52.7 | −37.0 | 78.6 |
0821−669 | DA9.8 | 16.8 | 4.5 | 4.4 | 18.0 |
0827+328 | DA6.9 | 4.1 | −45.4 | −6.6 | 46.1 |
0839−327 | DA5.3 | 38.2 | 26.6 | 4.6 | 46.8 |
0840−136 | DZ10.3 | 14.0 | −0.0 | −21.0 | 25.2 |
0843+358 | DZ6 | 7.6 | −5.5 | −13.6 | 16.5 |
0856+331 | DQ5.1 | 21.4 | 0.883 | −24.3 | 32.4 |
0912+536 | DCP7 | 21.0 | −42.5 | −24.3 | 53.3 |
0946+534 | DQ6.2 | 20.2 | −7.3 | −16.1 | 26.9 |
0955+247 | DA5.8 | 6.1 | −28.1 | −17.7 | 33.8 |
0955+247 | DA5.8 | 19.1 | −40.3 | −11.8 | 46.2 |
0959+149 | DC7 | −39.4 | 56.1 | −127.2 | 144.5 |
1009−184 | DZ7.8 | 36.1 | −8.8 | −26.4 | 45.6 |
1009−184 | DZ8.5 | 35.1 | −6.0 | −26.8 | 44.5 |
1012+083.1 | DA7.8 | 38.4 | 10.1 | −12.2 | 41.5 |
1019+637 | DA7.3 | −14.7 | 17.9 | 3.5 | 23.4 |
1033+714 | DC9 | 138.8 | −73.2 | −58.0 | 167.3 |
1036−204 | DQP10.2 | 36.0 | 17.9 | 20.7 | 45.2 |
1043−188 | DQ8.1 | 110.7 | −71.1 | −107.8 | 170.1 |
1055−072 | DA6.8 | 42.3 | −10.4 | −16.3 | 46.5 |
1105−048 | DA3.5 | −17.3 | −37.8 | −29.8 | 51.2 |
1116−470 | DC | 24.3 | −9.2 | −7.0 | 27.0 |
1121+216 | DA6.7 | 57.8 | −25.3 | −21.5 | 66.7 |
1124+595 | DA4.8 | −12.2 | 1.3 | 6.0 | 13.7 |
1132−325 | DC | −11.4 | 22.5 | 35.8 | 43.8 |
1134+300 | DA2.5 | 9.2 | −4.6 | −2.9 | 10.7 |
1142−645 | DQ6.4 | −52.1 | 25.3 | 6.6 | 58.3 |
1149−272 | DQ8.1 | 24.9 | −8.5 | −0.8 | 26.4 |
1202−232 | DAZ5.8 | 3.0 | 6.1 | 8.6 | 11.0 |
1208+576 | DAZ8.6 | −45.5 | −10.6 | 23.0 | 52.0 |
1214+032 | DA8.0 | 64.0 | −11.2 | 2.3 | 64.9 |
1223−659 | DA6.5 | 0.7 | 0.1 | −8.4 | 8.4 |
1236−495 | DA4.4 | 24.0 | −17.0 | −8.6 | 30.7 |
1241−798 | DC/DQ | 38.1 | −36.9 | 33.3 | 62.6 |
1242−105 | DA6.3 | 13.8 | −44.3 | 24.3 | 52.4 |
1257+037 | DA8.7 | −6.2 | −69.4 | −25.6 | 74.2 |
1309+853 | DAP9 | −16.3 | −1.3 | 15.4 | 22.5 |
1310+583 | DA4.8 | −16.3 | 6.7 | 5.7 | 18.5 |
1310−472 | DC11.9 | −133.6 | 81.4 | −51.5 | 164.8 |
1315−781 | DA | −23.5 | +26.4 | −32.3 | 47.8 |
1315−781 | DC8.8 | −30.2 | 34.0 | −41.7 | 61.7 |
1327−083 | DA3.7 | 49.2 | −76.6 | −7.4 | 91.3 |
1334+039 | DZ10.0 | 87.5 | −122.2 | 8.5 | 150.5 |
1337+705 | DAZ2.8 | 43.7 | −15.5 | 29.9 | 55.1 |
1339−340 | DA9.5 | 55.0 | 108.0 | 177.4 | 214.8 |
1344+572 | DA3.8 | 27.3 | 26.8 | 37.5 | 53.5 |
1344+106 | DAZ7.1 | 54.6 | −62.3 | 14.1 | 84.1 |
1345+238 | DA10.7 | 67.0 | −47.4 | 20.1 | 84.5 |
1350−090 | DAP5 | −21.7 | −23.0 | −23.2 | 39.2 |
1401+457 | DC19 | 16.9 | −27.6 | 5.5 | 32.9 |
1403+451 | DB | 3.9 | −2.1 | 1.2 | 4.5 |
1425−811 | DAV4.2 | −7.9 | −26.2 | −44.0 | 51.779 |
1436−781 | DA8.1 | 29.7 | −32.5 | 23.3 | 49.8 |
1444−174 | DC10.1 | 33.0 | −61.4 | 17.6 | 71.9 |
1529+141 | DA9.6 | −10.6 | −13.1 | −7.2 | 18.3 |
1532+129 | DA9.6 | −1.8 | −26.2 | 3.9 | 26.5 |
1538+333 | DA5.6 | 12.0 | −5.2 | 11.8 | 17.6 |
1544−377 | DA4.7 | 5.5 | −22.4 | 7.6 | 24.3 |
1609+135 | DA5.8 | −25.2 | −35.3 | −17.4 | 46.8 |
1620−391 | DA2 | −1.3 | 2.8 | −3.2 | 4.5 |
1625+093 | DA7.3 | −23.9 | −46.7 | −12.3 | 53.9 |
1626+368 | DZ5.5 | 35.1 | 15.5 | 35.6 | 52.3 |
1632+177 | DA5 | −2.7 | 2.3 | −5.3 | 6.4 |
1633+433 | DAZ7.7 | −12.7 | −4.2 | −13.9 | 19.4 |
1633+572 | DQ8.2 | 45.4 | −3.0 | 54.5 | 71.0 |
1639+537 | DAH6.7 | −3.6 | −19.1 | 8.9 | 21.4 |
1647+591 | DAV4.2 | −6.0 | −3.0 | −5.4 | 8.6 |
1655+215 | DA5.4 | −25.9 | −35.9 | −18.4 | 47.9 |
1655+215 | DA5.4 | −38.0 | −39.0 | −17.7 | 57.3 |
1658+440 | DAP1.7 | −6.6 | 33.0 | 28.2 | 43.9 |
1705+030 | DZ7.1 | −16.6 | −23.1 | −13.4 | 31.5 |
1729+371 | DA7.3 | −28.2 | 21.2 | 13.8 | 37.9 |
1748+708 | DQP9.0 | 5.8 | −10.8 | 34.2 | 36.3 |
1756+143 | DA9.0 | −36.3 | −86.3 | 49.1 | 105.7 |
1756+827 | DA7.1 | 8.8 | −30.3 | 106.4 | 111.0 |
1814+134 | DA9.5 | −47.7 | −72.0 | −8.6 | 86.8 |
1817−598 | DA5.8 | −15.1 | −22.9 | 2.3 | 27.6 |
1820+609 | DA10.5 | −9.8 | −9.0 | −19.7 | 23.7 |
1829+547 | DQP7.5 | 2.8 | −0.7 | 24.3 | 24.4 |
1840+042 | DA5.8 | 32.0 | −23.6 | 19.9 | 44.4 |
1900+705 | DAP4.5 | 6.7 | 5.5 | 6.0 | 10.5 |
1917+386 | DC7.9 | −5.2 | −5.7 | −8.6 | 11.6 |
1917−077 | DBQA5 | −5.4 | −6.4 | −0.5 | 8.4 |
1919+145 | DA3.5 | −4.2 | −5.0 | −0.7 | 6.6 |
1935+276 | DA4.5 | 16.0 | 10.2 | −31.7 | 37.0 |
1953−011 | DAP6.5 | −32.3 | −31.9 | 3.7 | 45.6 |
2002−110 | DA10.5 | 40.4 | 9.8 | −76.6 | 87.2 |
2007−303 | DA3.3 | −22.0 | −18.3 | 18.0 | 33.8 |
2008−600 | DC9.9 | −17.7 | −62.3 | −3.3 | 64.9 |
2008−799 | DA8.5 | 18.0 | −6.5 | −20.7 | 28.2 |
2011+065 | DQ7 | −64.2 | −24.8 | −17.0 | 70.9 |
2032+248 | DA2.5 | −36.9 | −24.9 | −2.8 | 44.7 |
2039−202 | DA2.5 | 15.9 | −3.9 | −30.9 | 35.0 |
2040−392 | DA4.5 | −13.6 | −25.2 | −0.3 | 28.7 |
2039−682 | DA3.1 | 0.698 | −17.8 | −9.9 | 20.4 |
2047+372 | DA4 | 13.4 | 5.5 | −1.4 | 14.6 |
2048+263 | DA9.7 | −37.6 | −16.2 | 11.8 | 42.7 |
2048+263 | DA9.7 | −79.5 | 104.1 | −13.4 | 131.7 |
2048−250 | DA6.6 | 5.3 | −13.6 | −20.0 | 24.8 |
2054−050 | DC10.9 | 31.1 | −11.4 | −54.1 | 63.5 |
2105−820 | DAP4.9 | 12.5 | −17.4 | 3.6 | 21.8 |
2117+539 | DA3.5 | −0.8 | 2.1 | 18.3 | 18.5 |
2115−560 | DA6 | 17.5 | −18.6 | −31.4 | 40.5 |
2118−388 | DC9.6 | 9.3 | −6.3 | −11.6 | 16.1 |
2126+734 | DA3.8 | 3.2 | 11.8 | −23.5 | 26.5 |
2133−135 | DA5.0 | 11.7 | −12.8 | −20.3 | 26.7 |
2138−332 | DZ7 | −15.0 | −8.3 | 8.4 | 19.1 |
2140+207 | DQ6.1 | −28.0 | −18.9 | −17.1 | 37.9 |
2149+021 | DA2.8 | −28.4 | −3.2 | −37.7 | 47.3 |
2151−015 | DA6 | −59.0 | 52.4 | −85.8 | 116.6 |
2154−512 | DQ7 | −12.4 | −22.4 | 9.9 | 27.5 |
2159−754 | DA5 | −27.6 | 7.6 | 18.5 | 34.1 |
2211−392 | DA8 | 57.8 | −43.7 | −44.5 | 85.1 |
2207+142 | DA6.6 | 21.1 | 83.8 | −45.7 | 97.7 |
2209−147 | DA6.6 | 54.9 | −21.5 | 30.4 | 66.3 |
2211−392 | DA8.1 | 46.6 | −43.1 | −61.1 | 88.2 |
2215+386 | DC10.6 | 48.7 | −7.1 | −20.6 | 53.3 |
2226−754 | DC9.9 | −0.5 | −88.5 | 71.5 | 113.8 |
2226−755 | DC12.1 | −0.5 | −88.5 | 71.5 | 113.8 |
2246+223 | DA4.7 | 42.4 | −10.4 | −16.9 | 46.8 |
2248+293 | DA9 | 111.2 | −29.8 | −43.0 | 122.9 |
2251−070 | DZ13 | 68.0 | −48.2 | −50.0 | 97.2 |
2253+054 | DA9 | 20.3 | −33.8 | −34.2 | 52.2 |
2311−068 | DQ6.8 | −45.3 | −1.7 | 7.4 | 45.9 |
2322+137 | DA10.7 | 3.2 | −0.5 | −0.3 | 3.2 |
2326+049 | DAZ4.4 | −31.6 | −2.1 | −1.3 | 31.7 |
2336−079 | DAZ4.6 | −5.8 | −13.4 | −5.9 | 15.8 |
2341+322 | DA4.0 | −18.8 | 5.6 | −0.4 | 19.6 |
2347+292 | DA9 | −18.3 | 2.7 | −57.2 | 60.1 |
2351−335 | DA5.7 | −62.0 | −22.5 | −38.2 | 76.2 |
2359−434 | DAP5.8 | 13.9 | −16.4 | −27.6 | 35.0 |
Table 4. Kinematical Statistics of Spectroscopic Subgroups within 25 pc
Type | Number | 〈U〉 | 〈V〉 | 〈W〉 | 〈T〉 |
---|---|---|---|---|---|
DA/DAZ | 133 | 2.5 | −18.4 | −5.8 | 48.9 |
33.9 | 33.8 | 28.6 | 33.0 | ||
DQ | 15 | −2.4 | −16.0 | −3.3 | 46.8 |
38.7 | 26.8 | 25.2 | 22.2 | ||
DZ | 14 | 2.0 | −21.5 | −14.0 | 47.0 |
35.0 | 20.4 | 25.3 | 25.0 | ||
DC | 26 | −4.1 | −27.0 | −7.8 | 66.6 |
46.7 | 49.8 | 41.3 | 50.8 | ||
DAP/DAH | 11 | 3.3 | −10.2 | 0.9 | 42.0 |
30.6 | 30.7 | 26.3 | 27.5 | ||
Mag.non-DA | 5 | 4.2 | −19.5 | −0.4 | 47.0 |
25.4 | 28.5 | 16.9 | 25.0 |
Download table as: ASCIITypeset image
If we take T > 150 km s−1 as the lower cutoff for halo space motions, then there are seven stars or 3% of the total sample that have possible halo Population II stars in extreme galactic orbits that could happen to be interlopers in the solar neighborhood. These objects are WD0747+482.1 (DC10.4; 156 km s−1), WD0747+482.2 (DC12.0; 156 km s−1), WD0959+149 (DC7; 144 km s−1), WD1310−472 (DC11.9; 166 km s−1), WD1334+039 (DA11.0; 151 km s−1), WD1339−340 (DA9.5; 215 km s−1), and WD1756+827 (DA6.9; 156 km s−1).
To further investigate the question of population membership, we point out that the assignment of reliable population membership for local WDs must involve not only the vector components of the space motion but also the cooling ages derived from their surface temperatures and total stellar age. In contrast, the population membership of main sequence stars utilizes kinematical characteristics as well as chemical abundance data, e.g., metallicity. Despite this difference in the way population membership is assigned for the two types of stars, it is useful to compare the velocity distribution of the WD sample in the U versus V velocity plane with the velocity distribution (velocity ellipsoids) characterizing a well-studied sample of main sequence stars (Chiba & Beers 2000; Sion et al. 2009).
However, a halo or thick disk member star cannot be identified on the basis of kinematic data alone. The candidate star must also have a total stellar age that is of the order of 12 billion years or older. The temperature of the six stars given in Table 1 indicates cooling ages well below 12 billion years. Thus, their space motions together with their total stellar ages lead to the conclusion that there is no clear evidence of halo WDs among the WDs within 25 pc of the Sun.
In Figure 2, we display the U versus V space velocity diagram for the 25 pc sample of WDs, with the assumption of zero radial velocity, relative to velocity ellipses for main sequence stars (Chiba & Beers 2000; see also Kawka & Vennes 2006; Sion et al. 2009). DA stars are denoted by closed circles, while non-DA stars are denoted by closed triangles. In the diagram, the 2σ velocity ellipse contour (denoted by the solid line) of the thin disk component is displayed, as well as the 2σ ellipse of the thick disk component (short-dashed line) and the 1σ contour of the halo component (long-dashed line). The vast majority of WDs lie within the thin disk, as expected for the local sample. However, we see that nearly equal small numbers of DAs and non-DAs lie outside the thin disk ellipsoid but within the thick disk ellipse, while three stars, two DAs and one non-DA, lie clearly within the halo velocity ellipse. Finally, one non-DA and two DA stars have anomalous velocities, placing them at very large positive V velocity components.
Figure 2. U vs. V space velocity diagram for the 25 pc sample of white dwarfs with the assumption of zero radial velocity. DA stars are denoted by closed circles, while non-DA stars are denoted by closed triangles. For comparison, three velocity ellipses for main sequence stars are shown following Chiba & Beers (2000): the 2σ velocity ellipse contour (solid line) of the thin disk component, the 2σ ellipse of the thick disk component (short-dashed line) and the 1σ contour of the halo component (long-dashed line).
Download figure:
Standard image High-resolution imageGiven the concentric, nested nature of the thin and thick disk velocity ellipses in the U − V plane, it is difficult to clearly disentangle the two populations. Age is a strong discriminate, and careful consideration of this together with the use of individual galactic orbits (see Pauli et al. 2006 and others) may help to better separate these populations.
4. DQ STARS WITHIN 25 pc
In Figure 3, we display the distribution of Teff for the 23 DQ WDs (upper panel) and 28 DC WDs (lower panel) within 25 pc. Although any comparison with the non-DA subgroups suffers from small number statistics, the number of DQ stars appears to peak at lower temperatures, as expected since the C2 molecular absorption bands strengthen with decreasing Teff. Although the number of stars in both distributions is small, it is noteworthy that the DC stars extend to even lower temperatures than the DQ stars. The previously known precipitous cutoff in the number of DQ stars at surface temperatures cooler than ∼6500 is seen in Figure 3. This real cutoff was first noted by Bergeron et al. (2001) and subsequently found in the much larger Sloan Digital Sky Survey (SDSS) sample of DQ stars by Dufour et al. (2005) and by Koester & Knist (2006).
Figure 3. Histogram of the number vs. surface temperature distribution function for all DQ white dwarfs (upper panel) and all DC white dwarfs (lower panel) within 25 pc of the Sun. Note the pileup of DC stars near the temperature at which the DQ stars show a real cutoff.
Download figure:
Standard image High-resolution imageReturning to the temperature distribution of 28 DC stars and 23 DQ stars, while mindful of small number statistics, it is nonetheless interesting that we see a large increase in the number of DC stars beginning at or near the temperature at which the DQ cutoff is seen. While some of these DC stars may show line features at high enough spectral resolution and sensitivity while other DCs may turn out to be hydrogen-dominated, we suggest the possibility that some of the 28 DCs within 25 pc could possibly be heretofore unidentified DQ stars with extreme pressure shifts that render their spectra unrecognizable. Alternatively, there may be some physical mechanism that removes carbon from the smallest optical depths in the atmosphere.
The peculiar DQ stars, the so-called C2H stars, appear at the low temperature end of the DQ distribution of Teff. Hall & Maxwell (2008) pointed out that the peculiar absorption features first identified as C2H in the spectra of cool (Teff < 6000 K) peculiar DQ stars were misidentified as hydrocarbon molecules, and instead are the extremely pressure-shifted Swan bands of C2. Kowalski (2010) showed that the distortion of Swan bands originates in the pressure-induced increase in the electronic transition energy between states involved in the transition. Unfortunately, the predicted Swan band shifts are too large compared to the observed ones. Thus, the need for further work in this area is indicated.
5. MAGNETIC WHITE DWARFS WITHIN 25 pc
The true incidence of magnetic WDs in a volume-limited survey remains an open question. Out to 25 pc, we count a total of 19 magnetic degenerates, among which there are 14 DA magnetics and 5 non-DA magnetics. Liebert et al. (2003) presented evidence that the true incidence of detected magnetism among field WDs at the level of ∼2 MG or greater, is at least 10%, and may be higher. The incidence of detected magnetism in our volume-limited sample is 8% but would be as high or higher than the Liebert et al. (2003) percentage if surveys of field strengths below 2 MG were carried out for our sample.
The relatively small number of magnetics within 25 pc prevents a determination of whether or not the field strength of a magnetic WD varies as a function of time (stellar age). For the same reason, we cannot say if the fraction of magnetic WDs is higher among cool star samples (e.g., samples of field WDs near the Sun) than among surveys of field WDs that extend out to greater volumes of space and hence sample hotter WDs. It remains an open question whether the number of magnetic WDs increases with decreasing Teff, luminosity, or cooling age. The answers to these questions must await volume-limited surveys sufficiently large in radius to encompass substantial numbers of hot WDs across a broader range of Teff.
6. COOL WHITE DWARFS WITH METALS
We count 26 cool WDs in the sample that exhibit absorption features due to accreted metals. This number excludes the DQ stars because although some may accrete metals, the carbon in their atmospheres is either primarily due to convective dredge-up (Dufour et al. 2005) or is primordial (Dufour et al. 2007). There has been no definitive explanation for why the DZ stars and DQ stars appear to have mutually exclusive spectra; almost no DQ stars reveal absorption features due to metals (other than carbon), while the DZ stars rarely exhibit absorption features due to carbon. It is unlikely that accreted metals may be easier to hide in DQ atmospheres due to increased opacity provided by carbon. We note that Weidemann & Koester (1989) analyzed a DZ star that contained carbon. More recently, Koester et al. (2011) reported a DQ star from the SDSS that reveals a decrease in flux at the blue end, which could be explained with calcium and possibly iron. It is also possible that the apparent dichotomy between DQ and DZ stars could be due to selection and small number statistics. Moreover, since the DQ stars require very deep convection zones to dredge up carbon, it is also possible that any accreted metals are diluted to such an extent that metal features are too weak to be detected.
Among these 26 WDs within 25 pc are 2 DZAs, 13 DAZs, and 11 DZ stars. For these objects, the most likely source of the accreted metals is from debris disks (Farihi et al. 2009 and references therein).
On this basis, we speculate that all 26 non-DAs with photospheric metals accreted from dust/debris disks of tidally disrupted asteroids, or in the case of DAZ stars, from volatile-rich matter such as comets. Thus, we speculate that at least 11% of the WDs within 25 pc have circumstellar debris disks with rocky, metallic debris and very likely descended from main sequence progenitors that had planetary systems. By comparison with frequencies of occurrence of exoplanets among Sun-like stars, Howard (2013) and Mayor et al. (2011) find that 15% of Sun-like stars host one or more planets with mass Msini = 3–30 Earth masses orbiting within 0.25 AU, and another 14% of Sun-like stars host planets with Msini = 1–3 Earth masses. The similarity between the frequency of occurrence of exoplanets and the fraction of WDs with metal lines within 25 pc is almost certainly just a coincidence since we do not know exactly how many of the WDs in the 25 pc sample have been observed with high resolution.
Among the 25 pc sample in Table 1, we have uncovered three WDs with IR excesses from WISE photometry that were previously unknown. This is suggestive of debris disks (Cox et al. 2014) associated with the three WDs.
7. PRELIMINARY TESTS OF COOL WHITE DWARF SPECTRAL EVOLUTION
The total space density of WDs within 25 pc remains unchanged from the 20 pc sample (Holberg et al. 2008b), while the completeness drops from 85% at 20 pc to ∼60% at 25 pc. The space density of WDs in Holberg et al. (2008b) is derived from the virtual completeness of the WDs within the core 13 pc sample. This can be determined in two ways: from a direct count of WDs within 13 pc, or by matching the slope of a log N – log (distance) plot to an expected slope of −3. The completeness estimates for the 20 pc and 25 pc samples are then simply computed with respect to the measured space density. Nevertheless, by considering the 20 pc sample, the nature of the incompleteness at 65% can be considered well characterized. We can use this knowledge to conduct a simple, preliminary, prospective test of WD spectral evolution to test the hypotheses that the ordinary DQ stars (i.e., excluding the class of hot DQs) are descendants of cooling DB stars (Koester et al. 1982; Wegner & Yackovich 1984).
The formation rate of DB stars can be estimated from the following expression:
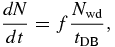
where f is the ratio of DB degenerates (Kleinman et al. 2004) to all other WD types within the observed range of Mbol for DB stars, Nwd is the local space density of WDs in the same range of Mbol as the DB stars, and tDB is the time spent by DB stars cooling within their observed range of Mbol. Nwd is obtained from a cool WD luminosity function.
The temperature range of the DB WDs is 30, 000 K > Teff > 12, 000 K, corresponding to a luminosity range −1.01 > Log(L/L☉) > −2.52. For DB stars, the local space density within the interval 12, 000 K < Teff < 30, 000 K is

and the parameter f = 20% for DB stars (Kleinman et al. 2004). The 20 pc sample of Holberg et al. (2008) contained no spectroscopically identified DB stars. Bergeron et al. (2011) conducted a comprehensive analysis of 108 DB stars, including spectroscopic distance estimates, finding four DBs within 25 pc. One, WD2147+280, has a trigonometric parallax that yields a distance of 35.7 ± 0.4 pc, which, as the authors point out, is difficult to reconcile with the estimated distance unless the spectroscopic gravity is reduced to 8.2, in which case it becomes consistent with the larger distance. After calculating distance uncertainties from the results of Bergeron et al. (2011), we included three of these stars, WD1542−275, WD2058+392, and WD2316−173, in our new local sample including appropriate distance uncertainties. Overall, Bergeron et al. found a DB space density of 5.15 × 10−5 pc−3 that gives an expected number of approximately four DB WDs in the 25 pc sample, which compares favorably with the three DB stars included in our sample. If we assume an average DB mass, MDB = 0.6 M☉, then the cooling timescale for a DB from 30,000 K to 12,000 K tDB ≈ 3.95 × 108 yr, according to the cooling models of Bergeron et al. (2011 and references therein).4 Hence, the formation rate of DB stars, dNDB/dt, is

Likewise for the formation rate of DQ stars from the following expression:
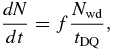
where f is the ratio of DQ degenerates to all other WD types within the observed range of Mbol for DQ stars, Nwd is the local space density of WDs in the same range of Mbol as the DQ stars, and tDQ is the time spent by DQ stars cooling within their observed range of Mbol. Nwd is obtained from a cool WD luminosity function.
The temperature range of the DQ stars is 12, 000 K > Teff > 5500 K, corresponding to a luminosity range of −2.52 > Log L/L☉ > −3.88. For the DQ stars, f = 9%. Assuming that DQ masses are Mwd = 0.6 M☉, the cooling time for a DQ through its interval of Mbol is tDQ = 3.6 × 109 yr. The local space density of DQs within the interval 12, 000 K > Teff > 5500 K is NDQ ≈ 2 × 10−3 DQs pc−3. Thus, the formation rate of DQ stars, dNDQ/dt, is dNDQ/dt = 4.5 × 10−14 pc−3 yr−1. The lower formation for the DQ stars relative to DBs presumably may reflect that not all cooling DB stars become DQ stars and may become DA stars either through accretion of volatile-rich tidally disrupted bodies, interstellar H, or diffusive float up of H as the DB cools. This very tentative result must be confirmed with larger, more complete samples of WDs.
Although this evolution test is only preliminary (given the uncertainties in the parameters used), it is nonetheless illustrative of the far-reaching potential of enlarging the volume of space around the Sun containing known WDs, thus increasing the sample size of WDs in each astrophysically important spectroscopic subclass including the magnetic degenerates as well as the DA, DB, DQ, DZ, and DC degenerates.
8. CONCLUSIONS
Our study has revealed the distribution of WD spectral types for 224 degenerate stars in a volume-limited sample out to 25 pc. We find the following characteristics of the sample.
- 1.There is little or no evidence of halo or thick disk component members within 25 pc, but seven degenerates with extraordinarily high space motions.
- 2.The sample includes a sizable number of DQ stars and cool (mostly DC and DZ) WDs. We note a possibly significant pileup of DC stars at the low temperature cutoff of the DQ stars.
- 3.The incidence of magnetic WDs within 25 pc is 8% of the total sample. This is close to the estimate of the true incidence of field WDs in the galactic disk by Liebert et al. (2003).
- 4.We carry out a preliminary test of one scenario of WD spectral evolution theory, namely, that all of the DQ stars (excluding the "hot" DQs) are the evolutionary descendants of the DB WDs. Within the uncertainties in the true space densities of DB stars and DQ stars, we find preliminary evidence that the formation rate of DQ stars is smaller than the DB rate, suggesting that not all DB stars evolve into DQ stars below 12,000 K.
- 5.We find no compelling evidence of any significant differences between the space motions of the various spectroscopic subtypes.
As this study indicates that the local WD population, with its high degree of completeness, provides an invaluable ground truth sampling of the WD population, particularly in the galactic disk. As such, it affords a number of ways to help characterize much larger, deeper, and more distant WD samples that are not volume limited, for example, those from the SDSS. Toward that end, compelling motivation now exists to both increase the completeness of the current 25 pc sample and extend it to a greater distance, thus providing a larger basic sample. Such efforts will also set the stage for a time during the next decade when the European Space Agency Gaia mission will make possible a virtually complete determination of all WD distances and proper motions (but not necessarily stellar spectra) out to ∼100 pc. Also within the same time frame, large surveys such as the Large Synoptic Survey Telescope will be sampling WD populations well into the halo.
This work is supported by NSF grant 1008845. We are grateful for several helpful comments and corrections from an anonymous referee. We thank John Debes for comments on the frequency of exoplanets around Sun-like stars. J.B.H. also wishes to acknowledge NASA Astrophysics Data Program grant NNX1OAD76. This research has made use of the White Dwarf Catalog maintained at Villanova University and the SIMBAD database, operated at CDS, Strasbourg, France.