Abstract
The anomalous Nernst effect (ANE), one of the thermomagnetic effects studied for a long time, is attracting attention again owing to the increased interest in spin caloritronics. Control of the ANE is an essential topic for not only clarifying the interplay among heat, spin, and charge but also application to high-efficiency energy-harvesting devices. In this review, various topics related to the modulation of the ANE are discussed and the possibility of methods for its thermoelectric application are discussed. Systematic studies of the material dependence of the ANE and the discovery of anisotropy in the ANE are reviewed. Enhancement of the ANE in granular thin films and control of the ANE in a ferromagnetic oxide thin film by electric field are also reported. This review provides physical knowledge of the modulation of the ANE and proposes several ways of effectively utilizing the ANE in the "hot" spin caloritronic field.
Export citation and abstract BibTeX RIS
The author hereby retracts the above article. Some contents in the article should not be included in the review article at the present moment. I reached the conclusion that this article should be retracted.
1. Introduction
Spintronics, a word coined from spin and electronics, is a research field mainly dealing with both charge and spin in electrons.1) This field has yielded several practical applications such as sensor heads in hard disk drives (HDDs) and magnetoresistive random access memory (MRAM).2,3) Recently, spin caloritronics has been established as an important field of research4,5) and numerous studies have been reported on the interplay between heat and spintronics such as the spin Seebeck effect (SEE),6) the spin-dependent Seebeck effect,7) and the spin Peltier effect (SPE).8) Some applications have been proposed, for instance, power generators, thermometers, and coolers, in spin caloritronics.4) The Nernst effect is also a well-known thermomagnetic phenomenon involving conversion from heat current into voltage.9) When a temperature gradient and a magnetic field normal to each other are applied in solids, a Nernst voltage is induced normal to both directions. This phenomenon can be induced by the Lorentz force on electrical charges in solids. In particular, the anomalous Nernst effect (ANE) is seen in ferromagnetic materials with a spontaneous magnetization, which is manifested as a voltage (V) equal to the cross product of the magnetization vector (M) and temperature gradient vector (∇T).10) The ANE can be induced without a magnetic field for ferromagnets; thus, this effect is thought to be applicable to actual devices. Hence, attention has been focused on this transverse effect in spin caloritronics.11–15) If the heat current is replaced by an electrical current (Jc), an analogous phenomenon, the anomalous Hall effect (AHE), is seen in ferromagnets as shown in Fig. 1.16–19) These two phenomena are thought to be induced by the scattering through the spin–orbit interaction, and they are associated by the phenomenological relation, that is, the Mott rule.20) Hence, the ANE and AHE have a close relationship, and a systematic investigation of the ANE and AHE for the same material helps to understand the fundamental and microscopic characteristics of both effects.12,21,22)
Download figure:
Standard image High-resolution imageFig. 1. Schematic images of (a) AHE and (b) ANE.
Download figure:
Standard image High-resolution imageWe have focused on the ANE for perpendicularly magnetized thin films. Chemically ordered (L10 type) FePt alloy thin films were fabricated by sputtering, and the ANE was measured at room temperature.23) Parameters related to the ANE, for instance, the anomalous Nernst coefficient (Qs), were experimentally evaluated for the FePt thin films. Qs is defined as follows:

where Exy, μ0, and Ms are the electric field across the temperature gradient, vacuum permeability, and saturation magnetization, respectively. The voltage due to the ANE in a nanometer-size spin Hall device was also simulated by the finite element method as shown in Fig. 2, in which a simulated temperature distribution was shown when an electrical current of 0.75 mA was applied in the Au bar. A diffused heat current flowed along the Au Hall bar and a Nernst voltage was induced between the two small circles at the edges of the FePt bar in the figure. Good agreement in the Nernst voltage between the experiment and the simulation was found even in nanoscale devices.
Fig. 2. Schematic model of multiterminal device (nanometer-size spin Hall device) used in the FEM calculation.
Download figure:
Standard image High-resolution imageThe ANE is also expected to be applicable to suitable devices such as thermoelectric conversion elements.24) We discovered that a chemically ordered (L10 type) MnGa alloy exhibits a strong ANE signal with the opposite sign to that of L10-FePt.25) Thermopile structures were fabricated that alternately employed the two materials (L10-FePt and L10-MnGa), and the ANE voltage was measured for the structures as shown in Fig. 3(a). Here, a magnetic field was applied perpendicular to the plane, a thermal gradient (∇T = 3.3 K/mm) was applied along in-plane direction, and the integrated voltage was measured between the edges of the thermopiles. Figure 3(b) presents measured voltages for two thermopiles with different numbers of piles. Clear hysteresis loops of the Nernst voltage plotted against the applied magnetic field were shown for both thermopiles. It was also found that the anomalous Nernst voltage increased in proportion to the number of piles, that is, the saturated voltage of the thermopile with nine piles was three times larger than that of the thermopile with three piles. Note that the widths of thermopiles in both devices are same, hence the temperature gradient provided for both devices was also the same. This result demonstrated the new concept of a thermoelectric conversion device using the ANE.
Download figure:
Standard image High-resolution imageFig. 3. (a) Schematic of thermoelectric conversion element with FePt/MnGa piles. (b) Magnetic field dependence of Nernst voltage in the thermopiles with three and nine piles.
Download figure:
Standard image High-resolution imageAs discussed above, it is indispensable to control properties of the ANE such as the magnitude and sign of the ANE signal at room temperature by various means to realize ANE-based applications. A few ways of enhancing the ANE signal have been reported so far. For instance, enhancement of the ANE in metallic multilayers such as Pt/Fe, Au/Fe, and Cu/Fe was reported, and the possibility of unconventional interface-induced thermoelectric conversion was proposed.26) In addition, significant enhancement of the ANE for ferromagnetic ultrathin metal films of Fe, Co, Ni, and permalloy was reported in a thickness-dependence study.27) Thus, the establishment of methods to control the ANE is presently an essential topic. In this review, the relationship between the magnetic anisotropy and ANE was studied for several epitaxial ordered alloy thin films with perpendicular magnetization to obtain a guiding principle for finding materials with a large ANE.28) The AHE of these materials, which also has a strong correlation with the spin–orbit interaction, was studied to compare its behavior with that of the ANE. Next, the strong anisotropy discovered in ferromagnetic nitride thin films is presented.29) This observation of the anisotropy in the ANE is the first report so far and is expected to help in tuning the magnitude of the ANE. On the other hand, granular films are expected to be utilized in spintronic devices because the magnetic properties and magnetotransport of granular films are completely different from those of pristine films. An analysis of the ANE in granular films with different compositions of metallic particles was performed,30) as reported in this review. Enhancement of the ANE for granular structures was observed. Finally, physical control of the magnitude of the ANE by an electric field is demonstrated in a manganese oxide thin film, which exhibits colossal magnetoresistance and is also a promising material for next-generation spintronics.31) By giving an overview of these results, principles to control the ANE and the possibility of its application in spintronic ferromagnetic thin films are discussed.
2. Experimental methods
Sputtering was mainly used to deposit magnetic thin films on MgO(001) single-crystal substrates in this study. An epitaxial FePt film (30 nm) was deposited by co-sputtering Fe and Pt targets at a substrate temperature of 500 °C. An epitaxial FePd film (30 nm) was deposited using an Fe–Pd alloy target at 450 °C. Epitaxial MnGa and Mn2Ga films (30 nm) were deposited using Mn–Ga alloy targets with corresponding compositions at 350 °C, and the films were annealed at 500 °C. An epitaxial Fe4N film (100 nm) was deposited by reactive magnetron nitride sputtering at room temperature, and the film was annealed by in situ infrared thermal treatment at 300 °C to promote the chemical ordering of N atoms. Cox–(MgO)1−x granular films (100 nm) with various atomic compositions (x) were deposited by co-sputtering Co and MgO targets at room temperature. A Co/Ni multilayer (12 nm) was deposited on a MgO(111) single-crystal substrate by alternate layer deposition at room temperature in a molecular beam epitaxy chamber. The stacking of the multilayer was [Co (0.2 nm)/Ni (0.4 nm)]×20. La0.7Ca0.3MnO3 (7 nm) was deposited on a SrTiO3(001) substrate using a sintered target in a KrF-pulsed laser deposition system.
Crystallographic structures of these films were analyzed by conventional X-ray diffraction, and the chemical long-range ordering parameter was evaluated. Magnetic properties were analyzed by a superconducting quantum interference device or a vibrating sample magnetometer, and uniaxial magnetic anisotropies (Ku) were estimated from magnetization curves under in-plane and out-of-plane magnetic fields.
Nernst and Hall effects of the films were measured in a physical properties measurement system with a superconducting magnet. One side of the sample edge was heated and ∇T produced in the films was used to measure the ANE. The electric field along the temperature gradient (Exx) was measured, and the Seebeck coefficient (Sxx) was calculated as

Exy was measured with a magnetic field perpendicular to the film, and the transverse Seebeck coefficient (Sxy), which directly expresses the magnitude of the Nernst effect, was defined as

The AHE was measured by applying a constant electrical current instead of the temperature gradient in the ANE measurement configuration. The electric field across the constant current density with a magnetic field perpendicular to the film was measured and the Hall resistivity (ρxy) was estimated. Then, the Hall conductivity (σxy) was calculated as

where ρxx denotes the electrical resistivity. For the measurement of the ANE with an electric field, the thin film was converted into a patterned Hall bar structure and the ANE was measured with the gate voltage on top of the Hall bar.
3. Results
3.1. Material dependence of ANE in perpendicularly magnetized ordered-alloy thin films
An ordered alloy is an alloy consisting of regularly and chemically ordered atoms in the crystal and known to exhibit distinct characteristics. In particular, some ferromagnetic ordered alloys exhibit large uniaxial magnetic anisotropy owing to their regular atomic arrangement. For instance, CuAu (L10)-type ordered alloys composed of a 3d transition element and a noble metal, such as FePt, FePd, CoPt, and CoPd, show extremely large uniaxial magnetic anisotropy and coercivity.32–35) From these characteristics, L10 alloys are expected to be superior materials for magnetic recording and permanent magnets. On the other hand, manganese-based L10 and Al3Ti (D022)-type ordered alloys such as MnGa and MnGe are also known to have large uniaxial magnetic anisotropy, whereas they have small saturation magnetization and small Gilbert damping constants.36–40) These characteristics are advantageous for application to spin transfer torque random access memory.
The uniaxial magnetic anisotropy of these ordered alloys dynamically changes with the combination of elements and the type of ordered structures; thus, ordered alloys are suitable materials for studying the relation between the ANE and the magnetic anisotropy. In this section, four different ordered alloy thin films with uniaxial magnetic anisotropy, L10-FePt, L10-FePd, L10-MnGa, and D022-Mn2Ga, were epitaxially fabricated, and the ANE and AHE were systematically investigated at room temperature. Here, the four samples were fabricated by applying each set of growth conditions to maximize Ku. A Co/Ni multilayer film with perpendicular magnetization was also fabricated as a reference sample even though it was not an ordered alloy. Hysteresis loops corresponding to the Nernst voltage of the films were observed even though the sign and magnitude of the ANE varied among the materials. The Ku dependence of the absolute value of the anomalous term of Sxy (|(Sxy)s|) for the different materials is shown in Fig. 4(a). |(Sxy)s| monotonically increased with Ku as seen in the figure. This indicates that the ANE increases roughly with the strength of the spin–orbit coupling in the ordered alloys employed in this study. The AHE of these films was concurrently investigated. The Ku dependence of the absolute value of the Hall conductivity |σxy| for the different materials is shown in Fig. 4(b), and a monotonic increase in |σxy| with Ku was seen, similarly to that for the ANE. From these tendencies of the ANE and AHE, it is supposed that ordered alloys with perpendicular magnetization have a strong spin–orbit interaction, and that the ANE and AHE of these materials ultimately have an intimate relationship. This provides a guideline for obtaining a large ANE, that is, the utilization of ordered materials with large magnetic anisotropy.
Download figure:
Standard image High-resolution imageFig. 4. (a) Material dependence of absolute value of anomalous term of transverse Seebeck coefficient (|(Sxy)s|) at 300 K as a function of Ku. (b) Material dependence of absolute value of Hall conductivity (|σxy|) at 300 K as a function of Ku.
Download figure:
Standard image High-resolution image3.2. Strong anisotropy of ANE in a ferromagnetic nitride thin film
Nitride alloys are also ferromagnetic ordered alloys. Magnetic properties of ferromagnetic nitride alloys such as V–N, Cr–N, Mn–N, Fe–N, and Co–N have been widely studied both theoretically and experimentally.41,42) Among others, γ'-type Fe4N is known as a ferromagnetic conductor with an anti-perovskite structure.43) This material has a conduction electron polarization of almost −100% and exhibits inverse tunneling magnetoresistance;44,45) thus, much effort has been devoted to clarifying its peculiar characteristics.46–48) Thermomagnetic properties of γ'-Fe4N have been barely studied so far, whereas its electrical conduction is attracting interest as noted above. Highly ordered γ'-Fe4N thin films were epitaxially fabricated, and both the ANE and AHE of the films were measured as reported in this section. In particular, the anisotropy in the film plane of the ANE and AHE at room temperature was intensively studied.
Figure 5(a) presents two ANE voltage loops with the two different temperature gradient directions. One can see the strong anisotropy in the magnitude of the ANE, that is, the Nernst voltage with ∇Tx ∥ [110] is almost double that with ∇Tx ∥ [100] even though ∇Tx is the same for the two configurations (∇Tx = 0.35 K/mm). This means that a larger Nernst voltage is induced in the transverse direction to the temperature gradient along the hard magnetization axis than along the easy magnetization axis. It is supposed that the Nernst angle of γ'-Fe4N has strong anisotropy, but no studies on the anisotropy in the Nernst effect have been reported. It is possible that the observed anisotropy in the present study originates from the strong hybridization between Fe and N atoms, which is attributable to the particular anti-perovskite structure. To investigate whether this anisotropy also emerges for electrical conductivity, the AHE of the same films was measured. The Hall voltage as a function of the applied magnetic field with the two different electrical current directions is shown in Fig. 5(b). No distinct anisotropy was observed for the Hall voltage with an electrical current of 0.1 mA. This suggests that the Hall angle is isotropic and that the observed anisotropy in the Nernst effect is not directly related to the electrical conductivity.
Download figure:
Standard image High-resolution imageFig. 5. (a) Nernst voltage of γ'-type Fe4N thin film at room temperature as a function of applied magnetic field with the different measurement configurations. (b) Hall voltage of γ'-type Fe4N thin film at room temperature as a function of applied magnetic field with the different measurement configurations.
Download figure:
Standard image High-resolution imageAs discussed, the ANE can be effectively controlled through the anisotropy by employing a material with a distinctive crystal structure. Moreover, γ'-Fe4N consists of Fe and N, which are elements with large Clarke numbers, hence this is also a valuable material from the aspect of the element strategy. Nitride materials might have more varied coordination numbers than widely used oxide materials, thus possibly having unknown functionalities. In general, they also have high electrical conductivity, high corrosion resistance, and satisfactory mechanical strength. The development of nitride spintronics is expected in the future.
3.3. Enhancement of ANE in granular thin films
A structure composed of particles dispersed in a matrix of another material is called a "granular system".49) In particular, in the spintronics field, granular structures with nanosize metallic particles in an insulating or organic matrix are often studied. For instance, enhanced magnetoresistance and spin accumulation, and novel magnetotransport and spin dynamics have been reported for granular films with ferromagnetic nanoparticles.50,51) Current-induced tunnel magnetoresistance due to spin accumulation has been observed even for a granular film with nonmagnetic nanoparticles.52) However, few studies on the thermomagnetic or thermoelectric properties of granular systems have been reported, although many studies have been reported in spintronics. In this study, the ANE of granular films that include Co nanoparticles embedded in MgO matrixes was investigated at room temperature in relation to the microstructure. Several granular films with the atomic composition of Co varying from 57 to 100 at. % were fabricated. As a result of transmission electron microscopy observation, it was clarified that these films include Co particles with a diameter of nm order within a MgO matrix.
The Co atomic composition dependence of Sxy estimated from the ANE measurements is shown in Fig. 6(a). Sxy remained almost constant below the composition of 80 at. %, whereas it clearly increased above 80 at. %. After it showed a maximum at 96 at. %, it started to decrease. This rapid change in Sxy might be due to the change in the microstructure. In other words, it is supposed that the electron transport in the granular system changes from the tunneling regime to the metallic transport regime with increasing Co composition because the average size and the distance between adjacent Co particles gradually change. This result showed the enhancement of Sxy for a few granular films compared with that for the Co film (Co: 100 at. %), and that it is possible to increase the ANE by adjusting the Co composition. Sxx for these films was also investigated simultaneously. It monotonically increased with the Co atomic composition (not shown here). The anomalous Nernst angle (θANE), which is the ratio of the ANE to the Seebeck effect, was evaluated by the following equation:

Figure 6(b) shows θANE as a function of the Co composition. θANE remained around 0.05–0.06 for granular films with the Co composition below 96 at. % with some scattering. Above 96 at. %, it suddenly decreased to 0.02. It is concluded from these results that all the granular films have larger anomalous Nernst angles than that of the Co film (Co: 100 at. %). The formation of a granular film is significantly advantageous for enhancing the anomalous Nernst angle. It was also proved from our recent study that the anomalous Hall angle (θAHE) of this granular system has a completely different tendency as a function of the Co composition from that of the anomalous Nernst angle reviewed in this study.30) That is, θAHE, which is the ratio of the AHE to the electrical resistivity, increased almost monotonically with the Co composition. Investigating the relation between both effects in relation to the electron scattering is expected to lead to the elucidation of the mechanism of the ANE enhancement of this granular system.
Download figure:
Standard image High-resolution imageFig. 6. (a) Co atomic composition dependence of Sxy for granular films that include Co nanoparticles embedded in the MgO matrixes. The dashed line shows Sxy for a Co film (Co: 100 at. %). (b) Co atomic composition dependence of θANE for granular films that include Co nanoparticles embedded in the MgO matrixes. The dashed line shows θANE of the Co film (Co: 100 at. %).
Download figure:
Standard image High-resolution image3.4. Control of ANE in a ferromagnetic oxide thin film by electric field
Recently, manganites have been attracting much attention in spin caloritronics owing to the wide possibility of utilizing the interplay between spin and thermoelectric transport phenomena.53) In addition, the electric field effects in manganites such as the control of magnetization, magnetic anisotropy, domain structure, and spin polarization are interesting.54–61) To apply these electric field effects in actual devices, low energy consumption is extremely important, and the ability to control the ANE by a small electric field in manganites is expected. Here, control of the ANE in La0.7Ca0.3MnO3 thin films using a field-effect gated device is demonstrated. Figure 7(a) shows the temperature dependence of the field-cooled magnetization of a La0.7Ca0.3MnO3 thin film. It was found that this film has a magnetic ordering temperature of around 180 K. ρxx for this film as a function of temperature was measured for different bipolar gate voltages as shown in Fig. 7(b). A clear metal-to-insulator transition (MIT) due to the double exchange interaction was observed at a temperature close to 180 K. When a positive gate voltage (+2 V) was applied, ρxx increased around the MIT, whereas ρxx decreased with a negative gate voltage (−2 V). The Nernst voltage as a function of the magnetic field was also measured with a constant in-plane thermal gradient at 130 K for the same gate voltages. Figure 7(c) shows the normalized Nernst voltage, which was obtained by subtracting the normal Nernst term from the measured Nernst voltage. The normalized Nernst voltage appeared to change with the gate voltage. This indicates that the ANE was modulated by the electric field. It is also concluded that a small electric field of 3.33 × 105 V/cm is enough to modulate the Nernst voltage. This is the first observation of the modulation of the ANE by an electric field. The work presented here is a demonstration of a low-power spintronic and spin-caloritronic device that can be used as low-field switching device.
Download figure:
Standard image High-resolution imageDownload figure:
Standard image High-resolution imageFig. 7. (a) Temperature dependence of the field-cooled magnetization of La0.7Ca0.3MnO3 thin film. The dashed line is a guide to the eyes. (b) Temperature dependence of ρxx for La0.7Ca0.3MnO3 thin film for different gate voltages. The dashed line shows the magnetic ordering temperature estimated from the magnetization. (c) Temperature dependence of normalized Nernst voltage of La0.7Ca0.3MnO3 thin film for different gate voltages.
Download figure:
Standard image High-resolution image4. Discussion
Several ways to control the ANE are discussed. They are considered to be classified into three cornerstone categories as a strategy to enhance the ANE as summarized in Fig. 8. One is the utilization of materials with the capability to control the ANE. As such materials, materials with a large spin–orbit interaction, which is the root of the ANE, materials with a large anisotropy, and materials which evoke the phenomenon to enhance the ANE may be useful. The combination of such talented materials may effectively increase the ANE. The second is the practical use of nanostructures. This cornerstone includes the employment of a granular system, a porous system, or a multilayer, which leads to the significant enhancement of the ANE compared with that in bulk materials or pristine films. The last one is the control of the ANE by an external field. The application of an electric field or a piezoelectric field can be useful as an energy-saving method for spintronics.
Fig. 8. Schematic image of strategy to control ANE.
Download figure:
Standard image High-resolution imageThe effective combination of these approaches can be employed to control the ANE as a strategy for ANE application. The heat current intersects at right angles to the line joining two voltage terminals in the ANE, whereas the heat current is parallel to the line in the Seebeck effect. This difference in the geometry is a major advantage of ANE application because the Nernst voltage can be extracted without being affected by the electrical current induced by the heat current, enabling the design of thermoelectric elements with a high degree of freedom. We believe that a thermoelectric device based on the ANE that can operate at room temperature will consequently be realized in the near future.
5. Conclusions
Various topics related to the modulation of the ANE have been discussed along with the possibility of methods for its thermoelectric application. The relationship between the magnetic anisotropy and ANE was studied for several epitaxial ordered alloy thin films with perpendicular magnetization to obtain a guiding principle for finding materials with a large ANE. The ANE and AHE monotonically increased with Ku regardless of the material. The strong anisotropy discovered in ferromagnetic nitride thin films was reported. It is possible that the observed anisotropy in the present study is induced by the strong hybridization between Fe and N atoms, which is attributable to the particular anti-perovskite structure. ANE analysis was conducted for granular films with different compositions of metallic particles. Enhancement of the ANE for granular structures was observed. Physical control of the magnitude of the ANE by an electric field was demonstrated in a manganese oxide thin film, which is the first observation of the modulation of the ANE by an electric field. This review provides physical knowledge of the modulation of the ANE and proposes several approaches for the effective utilization of the ANE in the hot field of spin caloritronics.
Acknowledgments
The authors are grateful to Mr. K. Hasegawa, Ms. S. Ohata, Dr. Y. Sakuraba, Mr. T. Kamada, Dr. T. Kubota, Dr. T. Kojima, Dr. K. Takanashi, Dr. S. Mizukami, Dr. T. Miyazaki, Dr. K. Uchida, Dr. E. Saitoh, Dr. S. Isogami, Dr. M. Tsunoda, Dr. T. Kawai, Dr. P. Sheng, and Dr. H. Sharma of Tohoku University and Dr. C. V. Tomy and Dr. A. Tulapurkar of Indian Institute of Technology Bombay for their help with measurements, analysis, and fruitful discussions. This research was partly supported by JST-PRESTO, JST-CREST (Grant No. JPMJCR1524), Grants-in-Aid for Scientific Research (S) (25220910) and Scientific Research (A) (17H01052) from the Japan Society for the Promotion of Science, the Center for Spintronics Research Network (CSRN), and Collaborative Research Center on Energy Materials (E-IMR).
Biographies
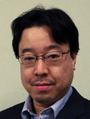
Masaki Mizuguchi is an associate professor in Institute for Materials Research, Tohoku University in Japan. He graduated from the University of Tokyo in 1998, and received his Ph.D. degree in Engineering from the University of Tokyo in 2003. After being an assistant professor at Osaka University, he obtained the present position in 2009. His major research fields are magnetic materials, spintronics, spin caloritronics, and multifunctional devices.