Abstract
Global industrialization and urbanization processes enabled a diverse cement production boom over the past three decades, as cement is the most important building construction material. Consequently, the cement industry is the second-largest industrial CO2 emitter (∼25% of global industrial CO2 emissions) globally. In this study, the Global Cement Emission Database, which encompasses anthropogenic CO2 emissions of individual production units worldwide for 1990–2019, was developed. A recently developed unit-level China Cement Emission Database was then applied to override China's data and the combination of two databases is used to reveal the unit characteristics of CO2 emissions and ages for global cement plants, assess large disparities in national and regional CO2 emissions, growth rates and developmental stages from 1990–2019, and identify key emerging countries of carbon emissions and commitment. This study finds that globally, CO2 emissions from the cement industry have increased from 0.86 Gt in 1990 to 2.46 Gt in 2019 (increasing by 186%). More importantly, the large CO2 emissions and the striking growth rates from those emerging countries, including most of the developing countries in the Asia region and the Middle East and Africa region, are clearly identified. For example, the Middle East and Africa, including mostly developing or underdeveloped countries, only represented 0.07 Gt CO2 in 1990 (8.4% of the total), in contrast to 0.26 Gt (10.4% of the total) CO2 in 2019, which is a 4.5% average growth rate during 1990–2019. Further, the intensive expansion of large and new facilities since 2005 in Asia and the Middle East and Africa has resulted in heavy commitment (90.1% of global commitment in 2019), and mitigation threats in the future considering their increasing emissions (the national annual growth rate can be up to >80%) and growing infrastructure construction (∼50% of clinker capacity operating ⩽10 years). Our results highlight the cement industry's development and young infrastructure in emerging economies; thus, future increasing cement demand and corresponding carbon commitment would pose great challenges to future decarbonization and climate change mitigation.
Export citation and abstract BibTeX RIS

Original content from this work may be used under the terms of the Creative Commons Attribution 4.0 license. Any further distribution of this work must maintain attribution to the author(s) and the title of the work, journal citation and DOI.
1. Introduction
Cement is an important building material that has been used since ancient times. The urbanization and modernization of human society has boosted cement demand in engineering and construction, and global cement production has surged from 1.2 Gt in 1990 to 4.1 Gt in 2019 (USGS 2021). However, the current cement industry is highly CO2-emitting (generally ∼0.59 tCO2 per ton of cement produced in 2020; IEA 2021) and is regarded as one of the most concerning sectors for CO2 emission quantification and future decarbonization in the context of prevailing climate governance (IPCC 2014). Following soaring cement demand and production, CO2 emissions from the global cement industry have climbed considerably since 2000, and the process emissions reached 1.57 Gt CO2 in 2019 (Guo et al 2021), accounting for ∼25% of global industrial CO2 emissions (Tong et al 2019). The International Energy Agency (IEA) has pointed out that there is an urgent need to lower the thermal intensity of the cement industry, and advanced low-carbon technologies (e.g. carbon capture, utilization and storage) should be applied to align with the Sustainable Development Scenario (IEA and WBCSD 2018). For feasible and efficient mitigation, more efforts are needed to understand the cement industry's historical CO2 emissions, underlying drivers of cement infrastructure evolutions, and emissions commitment (Tong et al 2019).
Some previous studies have comprehensively estimated global, regional, and national CO2 emissions from the cement industry (Keeling 1973, Marland and Rotty 1984, Boden et al 2014, Andrew 2018, 2019). Early studies relied on simplified time-invariant assumptions for key parameters (Keeling 1973, Marland and Rotty 1984), e.g. cement CaO content, and seldom considered country-specific characteristics (e.g. unit types, thermal intensities, and clicker-to-cement ratios). Recent studies have presented emission estimates under the Global Carbon Project framework (Le Quéré et al 2018, Friedlingstein et al 2020), including the collection of country-specific data, evaluation of key estimate parameters from multiple sources, and detailed emission analysis, but they focused on process emissions (Andrew 2018, 2019). Global emission inventories, such as the Emissions Database for Global Atmospheric Research and the Community Emissions Data System, encompass country-level CO2 emissions from the cement industry, which separate combustion and process emissions, but combustion emissions are usually reported as a total amount of non-metallic minerals (Hoesly et al 2018, Janssens-Maenhout et al 2019). The aforementioned studies provide important insights in emission magnitudes and trends at the regional or country level but limited detailed emission characteristics, such as production capacity development, emission change drivers and future commitment, which makes it difficult to identify different emission patterns among countries and reveal national mitigation urgency, difficulty, and future potential.
In-depth infrastructure transition studies are in need of unit-level emission quantification for the global cement industry. Although facility-level emission characteristics in China's cement industry have been analyzed (Liu et al 2021), a gap remains between country-level and unit-level analysis at the global scale. Filling the gap can strongly support targeted mitigation and promote our insightful understanding for future cement 'lock-in' emissions (Davis and Socolow 2014, Tong et al 2019). A unit-level database allows us to know the annual newly built capacity magnitudes, the historical trends of capacity growth, and the details of technological evolution, which are hardly provided by current country- or region-level databases. The derived information can better depict the historical evolution of the cement industry and facilitate future projection. Besides, the estimates of committed emissions also rely on unit-level information such as operating year, which would lay a solid foundation for revealing future climate risks.
In this work, a new global unit-based database, named the Global Cement Emission Database (GCED), is developed by integrating basic unit-based information on global clinker production lines from several international databases and estimating direct CO2 emissions (both combustion and process emissions) at the unit level. A recently developed unit-level China Cement Emission Database (CCED, Liu et al 2021) is then used to replace China's data in GCED as it is believed CCED represents China's cement industry more accurately (See details in section 2.2). Instead of integrating into the GCED, CCED is combined with GCED because GCED is a publically available database while information in CCED is not publically available due to restriction from the original data owners. The two databases encompass 5741 units in 4196 plants during 1990–2019 worldwide (table S1 available online at stacks.iop.org/ERL/17/044007/mmedia), including basic unit information, production information, and derived CO2 emissions. Based on the combination of these two unit-level databases, this paper first presents the global distribution of unit-based clinker capacity, age, and CO2 emissions as of 2019 to analyze the general differences in regional and national infrastructure and emission patterns. Cement-producing countries are then categorized into four representative regions with diverse emission characteristics: Asia, the Middle East and Africa, the Organization for Economic Co-operation and Development and the European Union (OECD and EU), and the rest of world. This study further presents region-specific trends in CO2 emissions by unit and age during 1990–2019 and reveals their underlying emission change drivers from new facilities. Finally, countries are ranked according to emissions, committed emissions, average remaining lifetimes of facilities, and emission growth rates in different years (i.e. 1990, 2005 and 2019) or stages (i.e. 1990–2000, 2000–2010 and 2010–2019), and the related characteristics of different regions and countries are analyzed.
2. Methods and data
2.1. Global cement emissions database (GCED)
In this work, a consistent time series of global unit-based cement emission database (GCED) is developed. GCED encompasses operating facilities for the period 1990–2019 worldwide and is a newly built emissions database of global cement plants, including infrastructure basic information, production information, and derived CO2 emissions.
This work starts by using the global detailed plant-level data obtained from the Global Cement Directory 2019 dataset (2020), including the basic operational information of production lines, such as company, plant name, physical address, start year of operation, total plant capacity, number of lines, and kiln type (i.e. dry, wet, semidry, semiwet and shaft) as of 2019. The comprehensive and reliable unit-level information of start year of operation, closed year, capacity, and unit type is further one-by-one supplemented according to the Industry About database (2019) and official company websites. In order to improve the accuracy of energy consumption estimates because different technologies have different energy intensities, unit-level specific technology information (i.e. dry with preheater and precalciner, dry with preheater without precalciner, dry without preheater, semi-wet/semi-dry, and wet/shaft) is again filled referring the company websites where available. For missing unit-level technology information, the gap is filled according to survey-based data of country- or region-level technology penetration rates from the World Business Council for Sustainable Development (WBCSD 2016, 2021), and assume the larger and newer units tend to have more advanced technology. Because geographical locations (exact latitudes and longitudes) are not included in the Global Cement Directory database, for the plants worldwide, their locations are obtained from the Industry About database where available. For the rest of plants missing geolocations, latitudes and longitudes are got by using Google Maps to map the physical address provided in the Global Cement Directory.
Unit-level process- and fuel-based CO2 emissions () are estimated using the following equation:

where i and k represent unit and country, respectively; represents unit-based emissions (kg);
represents cement production (t);
represents the clinker-to-cement ratio; F represents the fuel consumption (kJ);
represents the process-based emission factors (g/kg); and
represents the combustion-based emission factors (g/J). It is noted that only the direct emissions from cement production are estimated in this study; indirect emissions such as fuel use in the power plants due to electricity consumption and fuel use by vehicles for material transportation are not included (Liu et al
2021).
2.1.1. Activity rates
Because detailed activity data on cement and clinker production and fuel consumption from 1990–2019 for all the cement production units are not available, the annual unit-level cement and clinker production are therefore estimated by applying different methods. Country-level statistics of production and energy consumption for the period 1990–2019, which would be the constraints of unit-level information, are first collected. Global country-level cement production during 1990–2019 was obtained from the USGS (2021). Country-level clinker production is derived from collected clinker-to-cement ratio due to the data availability except China, whose cement production and clinker production can be obtained from National Bureau of Statistics, China Cement Association, and previous studies directly (National Bureau of Statistics 1991–2020a, China Cement Association 2001–2015, Xu et al 2012, 2014, Ministry of Industry and Information Technology of the People's Republic of China, 2016–2019, Gao et al 2017). Data on the national clinker-to-cement ratios are mainly adopted from the previous studies (Andrew 2018, 2019), which integrate the survey-based data at the regional and national level from the World Business Council for Sustainable Development (WBCSD 2016, 2021) and detail local information for key countries (e.g. India). It is noted that clinker-to-cement ratios varied over the past three decades and the significant changes such as in China and India has been observed (Guo et al 2021). Specifically, time-series clinker-to-cement ratios in India and other countries and regions are applied where available (e.g. the United States, EU28, and Latin America) for this study (WBCSD 2021). For the remaining countries, the ratio 0.86 of the Intergovernmental Panel on Climate Change (IPCC) default value is used due to data unavailability (2006).
Technology-based clinker production for each country (i.e. dry with preheater and precalciner, dry with preheater without precalciner, dry without preheater, semiwet/semidry and wet/shaft) are estimated according to the annual country- or regional-level production technology information for different kiln types for the 1990, 2000 and 2005–2019 derived from the WBCSD. A linear interpolation by kiln type with the available year is applied to fill time-series data gaps. Unit-level clinker production is a function of nameplate clinker capacity and annual capacity factor (that is, operating hours in a year), but of these, only installed cement capacity data are readily available, therefore, unit-level clinker capacity is proportionally converted from cement capacity according to the country-level clinker capacity (USGS 2021), and the unit-level real capacity factor or annual cement production from the Industry About database and official company websites is collected. For units missing real capacity factors, the simplifying assumption that annual average capacity factors of units are consistent at the country level is made for this study. In each year, unit-level clinker production from country-level clinker production is corrected by the equation:

where i, j, k, and y represent unit, technology, country and year, respectively. A represents clinker production, C represents clinker capacity, and CF represents annual capacity factor.
Thermal energy is consumed by the burning of fuels to heat the raw materials in cement kilns. Because country-level and unit-level fuel consumption for the cement industry is not available, the historical technology-based energy consumption intensities (i.e. GJ/ton-clinker) are first collected from the WBCSD (2021), which gathers detailed information for each plant in a common format and should be the most reliable, reflecting the actual consumption intensity (Oda et al 2012). The estimated global average energy consumption intensity for clinker production has decreased from 4.24 GJ/ton-clinker in 1990 to 3.46 GJ/ton-clinker in 2019 (WBCSD 2021). The energy consumption intensities significantly differ among different kiln types. For example, the global average energy intensities of dry with preheater and precalciner, dry with preheater without precalciner, dry without preheater, semiwet/semidry, and wet/shaft are 3.36, 3.50, 3.89, 4.16 and 5.36 GJ/ton-clinker for 2019, respectively. Similarly, there are also regional differences in average energy consumption intensity due to different penetration rates of kiln technologies. For example, energy consumption intensity in India, China, and the EU28 is relatively low compared to that in the U.S, such as a high diffusion rate for dry rotary kilns with 4-6-stage cyclone preheaters (and precalciners) and less usage of wet rotary kilns and vertical kilns (Oda et al 2012). Based on collected data and above-mentioned analysis, global time-series energy consumption intensity by kiln type from the WBCSD (covering 1990, 2000 and 2005–2018) are applied to the unit-level. The unit-level fuel consumption for combustion emission estimates is the product of clinker production and energy consumption intensity.
2.1.2. Emission factors
CO2 emission factors are estimated at the national level due to the difficulty of collecting unit-level data worldwide. The process-related CO2 emission is mainly from the decomposition of calcium carbonate (IEA 2016). For China, the CO2 emission factors are directly obtained from previous study (Shen et al 2016, Liu et al 2021). The CO2 emission factors are 519.66, 499.83, and 499.83 g kg clinker−1 for all dry, semiwet/semidry, and wet/shaft kilns, respectively. For other countries, emission factor data is collected from the literature (Andrew 2018, 2019), which is considered summarizing the best available information at present knowledge.
The combustion-related CO2 emission factors are estimated according to guidelines from the IPCC (2006) as presented in equation (3)
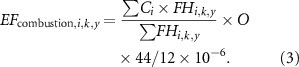
where i, k, and y represent the fuel type, year, and country, respectively; represents the combustion-based emission factors in g/J; C represents the carbon content of the different fuels in kgC/GJ;
represents the oxidation rate of fuels, and the carbon oxidation factor is assumed to be 1; and
is the energy consumption by fuel type from non-metallic mineral sources in GJ. The value of energy consumption is used to estimate the fuel-weighted carbon content due to data availability.
The energy consumption of each fuel type and country by year is from the IEA (2020) and calibrated for China from the China's Energy Statistical Yearbook 1990–2019 (National Bureau of Statistics 1991–2020b). It is noted that the energy consumption information for the cement industry is not counted separately in industry combustion sources from the IEA, therefore, the ratio of the heat amount for all fuel types from non-metallic mineral sources is estimated as a weight coefficient to calculate the combustion-related CO2 emission factors due to data availability. The IEA provides ∼40 fuel types for 1990–2018, and the 2019 data is extrapolated for each fuel type in each country.
2.2. China cement emission database (CCED)
CCED is an emission database for China's cement industry, which is developed based on intensive unit-based information on activity rates, production capacity, operation status for over 3100 clinker production lines (Liu et al 2021). Specifically, activity rates of coal consumptions in estimating combustion emissions at the unit level are calculated as the product of clinker production and annual energy intensity for the clinker production process. Unit-level clinker production from 1990 to 2019 are directly available in the CCED or derived from the national scale for the early years. The coal-use intensity of clinker production is derived through developing the linear regression as the energy efficiency in China's cement industry has improved markedly over the past three decades. The estimation of CO2 combustion emission factor follows the IPCC Guidelines, as presented in equation (3). The process CO2 emissions factor is collected from the previous study as that in the CCED (Shen et al 2016). In this way, the CCED improves the accuracy of the estimation of cement emissions, and provides a comprehensive view of the evolution of unit technologies for China's cement industry, which is used in the following analysis together with GCED.
2.3. Uncertainty analysis
The availability of activity rates, energy intensity, and emission factors could result in uncertainties (Streets et al 2003). Following the methodology in our previous studies for China's cement emissions (Liu et al 2021) and global power plant emissions (Tong et al 2018), a comprehensive analysis is performed for uncertainties in emissions estimated in this study at the national and unit levels using a Monte Carlo approach. The uncertainty is estimated by the 95% confidential interval (CI) around the central estimate from 10 000 Monte Carlo simulations with a specific probability distribution of input parameters, such as clinker-to-cement ratios, clinker production, energy intensity, emission factors and so on, which are obtained from previous studies (Liu et al 2015, 2021). Particularly, the ranges of parameter values related to CO2 emissions vary by country in part due to the quality of their statistical infrastructure. For example, input parameters of the clinker-to-cement ratio are in some cases obtained from previous studies without annual variation that represents larger uncertainties. Therefore, different statistical distributions and ranges of different parameters related to the CO2 emission estimates are derived in different ways by country and year to reflect the data availability and quality (See tables S2 and S3 in detail).
2.4. Committed CO2 emissions
Committed CO2 emissions represent the future 'lock-in' CO2 emissions of existing energy infrastructure within its lifetime (Tong et al 2019). In this study, the committed emissions from existing cement production lines for 2019 are estimated following a previous approach (Davis and Socolow 2014, Tong et al 2019). Specifically, commitment accounting requires the following information for each unit: (a) the year when the unit began operating, (b) the expected operating lifetime of the unit, (c) the annual emissions from the unit. The start year of operation and annual emissions at the unit level is directly from the two databases of GCED and CCED, and it is assumed in this study the unit will be operating as the same as that in 2019 (i.e. emission intensity and capacity factor) until retirement. Consistent with previous studies, a typical lifetime of 40 years is assumed for all units regardless of differences in country, kiln type, and operating year. The units that are operating beyond the assumed lifetime in 2019 are retired over the next 5 years to avoid abrupt changes. In this way, national committed emissions are estimated from the units' commitment.
3. Results
3.1. Contrasting trends in regional CO2 emissions during 1990–2019
Figure 1 shows the global cement plant distributions of clinker capacity, CO2 emissions, and age as of 2019. China, India and some other developing regions, such as Southeast Asia and the Middle East, have a large number of high CO2-emitting young and large infrastructure, which reflects their current dominant cement production (figure S1) and large unit expansion in recent decades. Some underdeveloped or developing regions, such as sub-Saharan Africa and Central Asia, also have young fleets but relatively small capacities and emissions, which indicates they are in earlier developmental stages. The unit features of developed regions, such as the United States and EU, are quite different with most of units operating over 30–40 years and relatively low emissions. Other regions, such as South America, have a mixture of small and large facilities across different ages. These global distributions clearly reveal the distinct emission patterns among countries at different developmental stages, which calls for further insights.
Figure 1. Maps of global cement plants worldwide. (a) CO2 emissions and (b) age distribution as of 2019 by plant clinker capacity (classified into five groups: <0.6 Mt, 0.6–1.2 Mt, 1.2–1.8 Mt, 1.8–2.4 Mt, and ⩾2.4 Mt).
Download figure:
Standard image High-resolution imageTo clarify the national differences in emission patterns, figure 2 presents national CO2 emission changes versus the annual average emission growth rates during 1990–2019. Both CO2 emissions (combustion and process CO2 emissions are analyzed together in this study, figure S2) and growth rates vary by several magnitudes, but similar emission or growth features are also observed. Therefore, these countries are grouped into four representative regions (Asia, the Middle East and Africa, OECD and EU, and the rest of world), by comprehensively considering their geographic locations, CO2 emission changes and growth rates, socioeconomic representativeness, and emission contributions. Asia is mainly dominated by countries with large CO2 emission increase and/or a rapid growth rate, and most of them are developing countries, with an average GDP per capita of 5436.8 $USD in 2019 (World Bank 2019). The Middle East and Africa, including mostly developing or underdeveloped countries, has relatively small emission increase compared to Asia but the growth is remarkable. Specifically, most countries in both Asia and the Middle East and Africa can be identified as emerging countries in the cement industry because of their rapid emission growth during 1990–2019, which largely shapes the industry's developmental patterns. The OECD and EU, the majority of which are developed countries (average GDP per capita of over 38 806.1 $USD in 2019), is characterized by stable CO2 emissions over years. The rest of world also has small CO2 emission changes accompanied by similar trends with those of the OECD and EU, except several countries with obvious emission growths. This region contains a mixture of rapidly developing countries (e.g. Brazil) and more developed countries (e.g. Russia).
Figure 2. CO2 emission changes and annaul average growth rates during 1990–2019 by country. The y-axis and x-axis demonstrate CO2 emission changes from 1990 to 2019 and the emission growth rate during 1990–2019 (global average growth rate 3.7%) by country, respectively. The size of the plots respresents the GDP per capita as of 2019, and the color of the plots respresents the four defined regions. Noted that the combined log (>1 and <−1) and equidistant (−1 to 1) y-axis are used to represent several mangtitudes of emissions changes.
Download figure:
Standard image High-resolution imageOverall, the past three decades have witnessed a soaring emission increase by 1.9 times of the global cement industry (0.86–2.46 Gt), but the emission patterns varied considerably worldwide and were significantly shaped by the surging emissions from the emerging countries. Figure 3 shows the regional contrast emission magnitudes and trends in the past three decades. The Asian countries showed an emission increase by 5.3 times during 1990–2019 and emitted 70.3% (1.73 Gt) of the total CO2 emissions from the cement industry in 2019 compared to only 32.1% (0.28 Gt) in 1990, which is consistent with their increased cement production (figure S3). The dramatical growth of this region was represented and led by the development of China and India. With rapid urbanization and industrialization, China's CO2 emissions from the cement industry increased by 7.2 times (7.5%/year) from 0.15 to 1.24 Gt during 1990–2019, accounting for 50.3% of global emissions in 2019. However, the emissions from China have remained stable since 2014 following the peak cement demand (see figure S1), reflecting the demand saturation associated with socioeconomic development. The reason for the slight increase in emissions since 2014 is because the clinker-to-cement ratio increased slightly (figures S4 and S5). CO2 emissions in India also increased considerably by 4.4 times (6.0%/year) and climbed to 0.22 Gt in 2019 but have not reached a zenith. Considering India's growing economy and large population, the CO2 emissions are expected to keep increasing with infrastructure expansion in the near future. Similar to China and India, the other countries in Asia showed an emission increase by 2.3 times during 1990–2019 and contributed 11.2% (0.27 Gt) of the total CO2 emissions in 2019. Due to their large emissions and rapid growth, the Asian countries should be the ones most targeted for future emission mitigation. The Middle East and Africa contributed to 10.4% of the total CO2 emissions in 2019, and the emissions climbed 2.6 times in the past 30 years, reflecting the great potential as a future emission hotspot. Thus, mitigation strategy should be proposed and adopted in advance, e.g. expansion of more energy-efficient technologies and evaluation of Carbon Capture and Storage (CCS) applications. The emerging countries in Asia and the Middle East and Africa together represent the strong drivers of CO2 emission increase in the past.
Figure 3. Trends in CO2 emissions from the global cement industry by region during 1990–2019.
Download figure:
Standard image High-resolution imageIn contrast with the emerging countries mentioned above, the OECD and EU showed steady emissions (−0.3% growth rates per year) during 1990–2019, contributing to 14.6% of total CO2 emissions as of 2019. As a representative of this region, the United States showed a slow growth rate (0.2% per year), and its contribution to total CO2 emissions was 2.5% in 2019 compared to 6.7% in 1990. The rest of world is the least concerning one for current emission mitigation due to its low emissions and slow or negative growth. Overall, the emission changes were different among the regions in the past 30 years, which indicates complex factors such as demand, different developmental stages and technologies. Our decomposition shows that production growth was the dominant driver in all the regions (figure S6). The decrease in the clicker-to-cement ratio, evolution of cement production technology and decrease in energy intensity can slightly offset the CO2 emission increase in Asia, especially during 2000–2010.
3.2. Regional emission characteristics by size and age
Figure 4 shows the CO2 emissions contributed by unit clinker capacity interval in 1990, 2005 and 2019 for each region. Overall, CO2 emissions shifting from small- (i.e. clinker capacity < 0.6 Mt per year) to medium- (0.6–1.2 Mt per year) and large-size capacity (≥1.2 Mt per year) units were remarkable in both Asia and the Middle East and Africa during 1990–2019. In 1990, emissions of Asia were mainly contributed by small-size units (73.8%). Although some larger units (0.6–1.6 Mt per year) were built during 1990–2005, the dominance of small-size units was still marked in 2005 (51.1%), which reflects the active construction of small-size semiwet/semidry or wet/shaft kilns in the early developmental stage (Liu et al 2021). After 2005, large-capacity dry process units with preheater and precalciner rapidly expanded in Asian countries like China and gradually replaced outdated small-size units. In 2019, 63.4% of CO2 emissions in the Asia region were contributed by large-size units, while small units accounted for merely 7.1%. The technological shift was driven by the soaring cement demand and energy-saving requirement there, which called for construction of large-scale plants with high efficiency. The emission changes in the Middle East and Africa were largely similar to those of Asia, but showed hysteresis to some extent. Small-size units dominated the emissions in 1990 (accounting for 65.6%) and accompanied by emission increase of medium-size units by 2005 (small-size units accounting for 39.9%). During 2005–2019, the emissions from large-capacity units considerably climbed, but meanwhile small- and medium-size units did not stop their emission increase. The small-, medium-, and large-size units accounted for 24.6%, 41.5%, and 33.9% of CO2 emissions in the Middle East and Africa as of 2019, respectively. This points to the mixed development of different kiln types and the relative immature developmental stage of the cement industry compared to most Asian countries. On the whole, although the progress varied, the CO2 emission contributions were gradually shifted to larger units in the emerging countries.
Figure 4. Clinker-capacity-based distributions of CO2 emissions in the year of 1990, 2005 and 2019. (a) Asia, (b) Milddle East and Africa, (c) OECD and EU, (d) the rest of world.
Download figure:
Standard image High-resolution imageChanges of CO2 emissions by unit size in the OECD and EU and the rest of world are significantly different. The emissions from small-size units kept shrinking in the OECD and EU, while those from medium- and large-size units slightly increased. This reflects the phase-out of some small-size inefficient units by policy or market competition in developed countries, accompanied by small-scale expansion of new modern plants to balance the supply. The emissions from small-size units in the rest of world also considerably decreased, but the emissions from larger units maintained steady. The outdated small plants were also eliminated, but new facility construction is more inactive there.
In addition to unit size, the CO2 emission shares of units in different age intervals also reveal the varying development characteristics (figures 5 and S7). The emission shares of units below 10 years old in Asia increased sharply after 2000 with a peak (72.6%) around 2010, and then gradually declined. The increasing trend accorded with the large-scale expansion of dry process units with preheater and precalciner (Liu et al 2021), and the share decrease later was mainly attributed to China's demand saturation and associated slowdown of new unit construction. However, a rebound of the emission shares of young units may be witnessed because of the continuing new construction in India and Vietnam. As for the Middle East and Africa, the contributions from young units below 15 years old increased after 2000 and have not seen obvious decrease, reflecting continuously demand growth and ongoing infrastructure expansion. In contrast with the emerging countries, the OECD and EU is characterized by the steady emission share increases of units over 30 years old, although new units were also built at small scale, reflected by the relatively constant contributions from young units. The shares of young units increased in the rest of world after 2000, but meanwhile the contributions from units above 30 years old also slightly climbed, which is attributed to the mixture of cement industry evolution in both developed and developing countries. Overall, the time-variant emissions by unit capacity and age both reveal regional emission patterns and shifts in detail, indicating various underlying influencing factors, such as demand evolution, technology transformation, and structural optimization.
Figure 5. Shares of CO2 emissions by unit age during 1990–2019. (a) Asia, (b) Milddle East and Africa, (c) OECD and EU, (d) the rest of world.
Download figure:
Standard image High-resolution image3.3. Key CO2-emitting countries
The top panel of figure 6 (figures 6(a)–(c)) shows the top 12 contributors to annual CO2 emissions in 1990, 2005 and 2019. In 1990, seven top CO2-emitting countries were from the OECD and EU, while only four countries remained in 2019 accompanied by an overall ranking decline. The CO2 emissions from the top countries belonging to the OECD and EU totally accounted for 45.8% of top-country emissions in 1990, and decreased considerably to 20.4% and 9.5% in 2005 and 2019, respectively. In contrast, China remained the top contributor, and India became the second-largest contributor, with more countries from Asia and the Middle East and Africa entering the top ranking. The new top contributors are mostly located in Southeast Asia and the Middle East, consistent with the rapid urbanization and infrastructure expansion in these areas. In 2019, CO2 emissions from China, India and other top emerging countries reached 1.7 Gt (i.e. located in Asia and Middle East and Africa), while the values were merely 0.19 Gt and 0.87 Gt in 1990 and 2005, respectively.
Figure 6. Summary of emissions and growth rates in the global cement industry. This figure shows the top 12 countries in terms of CO2 emissions (a)–(c), committed emissions (d)–(f), and average remaining lifetimes under the assumption of 40 year lifetime (g)–(i) in 1990, 2005 and 2019, as well as CO2 emission growth rates during 1990–2000, 2000–2010, and 2010–2009 (j)–(l). The country names are color-coded based on the four regions. Note that some values are labeled because they are beyond the maximum values of axis scales.
Download figure:
Standard image High-resolution imageThe changes in top countries are similar to annual emissions but more striking when considering committed emissions. The top CO2-committing countries from the OECD and EU committed a total of 4.0 Gt CO2 emissions in 1990 (figures 6(d)–(f)), but owing to little or small-scale unit expansion, only the United States and Turkey remained in the top rank in 2019, with 1.2 Gt CO2 commitment. In contrast, China, India and other emerging countries continuously expanded newly built units, embodied by the dominance of these countries in the ranking of average unit remaining lifetimes (figures 6(g)–(i)). The units can still survive for 20–40 years on average, which serves as an 'amplifier' for CO2 commitment (figure S8). By virtue of growing new infrastructure, the CO2 commitments of top CO2-committing emerging countries have increased from 6.2 Gt in 1990 to 47.6 Gt in 2019, among which China alone contributed 77.9% (37.1 Gt, figures 6(d)–(f)). Vietnam has climbed to the third-largest contributor, emitting 69.6 Mt CO2 in 2019 and committing 1.9 Gt CO2 emissions. Thus, mitigation is much more challenging for emerging countries, such as Vietnam with a large and young cement industry.
Finally, the CO2 emission growth rates by country are ranked (figures 6(j)–(l)). Interestingly, the ranks were dominated by underdeveloped or developing emerging countries in sub-Saharan Africa, Southeast Asia and Central Asia. The growth rate can exceed 20% per year and even approach 40% per year or more for some countries, and the cement demands in these areas are expected to continue growing in the next few decades, which indicates their great chance to play more dominant roles in the future.
4. Discussion
In this study, the multi-scale (region-, nation- and unit-level) changes of capacity, age, and CO2 emissions during 1990–2019 are explored by applying the combined two databases of the newly built GCED and recently developed CCED. Our study reveals the striking CO2 emission growths from the global cement industry during 1990–2019 driven by new facilities in emerging countries located in the Asia region and the Middle East and Africa region, and points to the current CO2 commitment risk there due to the large young fleets of cement production facilities. Asia has grown into a major contributor to current cement production, CO2 emissions, and committed emissions after 30 years' tremendous infrastructure expansion, led and represented by China, India and Vietnam. Despite much smaller emission shares compared to the dominant region now, the Middle East and Africa region has experienced surging emission growth and is expected to keep the increasing trend. The cement industry there should be closely tracked due to its great potential to produce future emission hotspots, and targeted mitigation strategies can probably be proposed and adopted in advance. Overall, the new facility expansion in emerging countries largely shaped the CO2 emission growth pattern in the past 30 years, and the expansion is still ongoing, continuously posing threats to climate goals and calling for timely and effective mitigation.
The unit-based cement emission databases successfully fill the country-to-facility emission gap, although they are subject to uncertainties and limitations. For example, unit-level activity estimates for the 1990s and 2000s were considered to be more uncertain because of incomplete unit-level information for the early years (figure S9). Clinker-to-cement ratio, technology information and thermal consumption data from the WBCSD, which are presented only in groups of countries, are still limited, although it is the best available information at present. The lack of local measurements ofCO2 emission factors and the assumption of combustion-related CO2 emission factors with the ratio of the heat amount for all fuel types from non-metallic mineral sources would introduce uncertainties. This study does not consider indirect electricity emissions, which are reported as 10% of the total emissions from the cement industry.
Regardless, our combined databases can reflect historical technological changes and transitions at unit level, which promotes our understanding of the cement industry's evolution and highlights the soaring growths of emerging countries driven by new facilities. This data-driven assessment adds important insights to policy-relevant discussions of climate change mitigation for the global cement industry. Decision makers in governments and the industry should not only formulate detailed policies and take 'point-to-point' actions on climate mitigation and future carbon 'unlock' especially in those identified dominant emerging countries, but also pay more attention to those 'small but growing' economies and find effective measures for balancing the potential contradiction between the development inertia and the low-carbon transition in the cement industry in the context of rapid economic development.
Acknowledgments
This work was supported by the National Natural Science Foundation of China (Grant Nos. 41921005 and 41625020) and the Energy Foundation (G-2009-32416).
Data availability statement
The company name, plant name, plant location, number of cement production units, combustion and process CO2 emissions at the unit-level contained in the GCED are available at: http://gidmodel.org.cn/dataset-gced. Other information at unit-level is obtained from commercial databases and is not publicly available. For the database CCED, aggregated information is available at: http://meicmodel.org/dataset-cced.html. Unit-level information is not publicly available due to restriction from data providers.
Author contributions
Q Z and D T designed the study. C C and D T performed the dataset development and emission analyses with support from R X, X Q, J C, J L, B Z and L Y on analytical approaches. C C and D T interpreted the data. R X, D T and C C wrote the paper with input from all co-authors.
Conflict of interest
The authors declare no competing interests.