Abstract
Severe acute respiratory syndrome coronavirus 2 (SARS-CoV-2) emerged in late 2019 and spread globally to cause severe damage to public health and economics worldwide as coronavirus disease 2019 (COVID-19). A deep ultraviolet light-emitting diode (DUV-LED) would be a promising candidate for the inactivation technology of SARS-CoV-2 to mitigate the spread of COVID-19. The DUV-LED has the potential to provide energy-efficient and contact-less virus inactivation technology with no residual substances after treatment, little effect on targets and the applicability of various viruses, including virus variants. This paper introduces an overview of the virus inactivation technology using DUV-LED and discusses the effect of the DUV-LED irradiation on the SARS-CoV-2.
Export citation and abstract BibTeX RIS
1. Introduction
Severe acute respiratory syndrome coronavirus 2 (SARS-CoV-2), causing coronavirus disease 2019 (COVID-19), is emerged in late 2019 and spread globally. 1–3) The COVID-19 pandemic has a significant impact on the well-being of people and nations worldwide with significant public health, economic, social and safety implications. Furthermore, there is concern that the effects will be prolonged in combination with the outbreak of SARS-CoV-2 variants and other seasonal infectious diseases. 4–7)
Several preventive measures against SARS-CoV-2 have been performed worldwide, such as vaccination, containment, social distancing, wearing face masks and washing hands; however, it is unpredictable whether various activities such as medical care, education, economy, and society can be back to normal. 6,8–17) Therefore, it is still required to perform multifaceted and multiplex countermeasures against the COVID-19.
This paper outlines an inactivation method of SARS-CoV-2 using deep ultraviolet (DUV) light-emitting diode (LED), one of the direct and effective preventive measures for COVID-19. 18–20) Virus inactivation technology using DUV light has several advantages: low energy cost, no residual substances after treatment, little effect on the target object, contact-less treatment, and effectiveness for most viruses, including variants. 18–33) Especially, DUV-LED is an attractive light source among DUV light sources because of its compact, lightweight, power-effective, and environmentally friendly (mercury-free) features. 34) In this paper, we review the fundamentals of the inactivation of viruses using DUV light and the effectiveness of DUV-LED on SARS-CoV-2 inactivation.
2. Novel coronavirus: SARS-CoV-2
2.1. Virological features
SARS-CoV-2 is classified as a severe acute respiratory syndrome-related coronavirus, genus Betacoronavirus, family Coronaviridae, suborder Cornidovirineae, order Nidovirales, and realm Riboviria. 35) Some other human respiratory coronaviruses include HCoV-229E, HCoV-NL63, HCoV-OC43, HCoV-HKU1, SARS-CoV and MERS-CoV.
SARS-CoV-2 is a positive-sense, single-stranded RNA virus with an envelope. The genome RNA of SARS-CoV-2 is approximately 30 kb in length. 36) The shape is a sphere with a diameter of about 100 nm, and the virus surface exhibits a crown-like appearance (Fig. 1). A lipid bilayer membrane surrounds the SARS-CoV-2, namely envelope. On the envelope, there are several proteins such as spike (S) protein, envelope (E) protein, membrane (M) protein, which play essential roles in attachment, fusion, and invasion to host cells. RNA is encapsidated by nucleocapsid (N) proteins and surrounded by the envelope. This RNA has the genetic information of the virus and essentially contributes to viral replication after infection.
Fig. 1. (Color online) Structure of SARS-CoV-2.
Download figure:
Standard image High-resolution image2.2. Fundamental mechanism of SARS-CoV-2 infection
The fundamental mechanism of SARS-CoV-2 infection is as follows (Fig. 2). 37,38) SARS-CoV-2 is most readily transmitted by respiratory droplets when an infected person speaks, coughs or sneezes within a short distance. 39,40) Another possible pathway is the contact of hands to mouth, eyes or nostrils just after a person touches a surface or object contaminated with SARS-CoV-2. 41) After the invasion into the human body, SARS-CoV-2 recognizes host cells by binding the S protein of the virus to the membrane protein (ACE2) of the host cells. 42–45) The host cells of the SARS-CoV-2 are mainly mucosal epithelial cells such as the airway, lungs, and intestinal tract. 46,47) The attached SARS-CoV-2 enters the host cell through receptor-mediated endocytosis or membrane fusion. 48) After the viral entry, the SARS-CoV-2 releases viral RNA containing the genetic information into the cytoplasm of the host cells. The replication of viral RNAs and the synthesis of viral proteins are occurred by utilizing the resources of the host cells. The synthesized viral proteins are assembled with the replicated viral RNAs to be newly replicated viruses. The lipids of the envelope of the replicated viruses are obtained from the host cell. After the viral assembly, the replicated viruses are released from the host cells. The repeat occurrence of this viral replication causes functional changes and damage of the host cells, leading to infectious diseases such as severe respiratory diseases. 49,50)
Fig. 2. (Color online) Overview of the infection process of SARS-CoV-2.
Download figure:
Standard image High-resolution image3. Inactivation of SARS-CoV-2 by DUV-LED
3.1. How to inactivate the SARS-CoV-2?
A countermeasure against COVID-19 is to make SARS-CoV-2 unable to replicate by inhibiting any step of the replication process described in Sect. 2. For example, social distancing and wearing masks, widely employed worldwide, are measures to avoid contact between SARS-CoV-2 and host cells existing such at mouth, eye and nostril. 39,51) Alcoholic treatment will disrupt the viral envelope, leading to avoiding attachment, fusion and invasion to host cells. 52) Alternatively, even if the SARS-CoV-2 invades into host cells, the viruses will not replicate if their replication ability is inhibited, namely, inactivation of viruses. This viral inactivation can also be an effective countermeasure against COVID-19.
3.2. Fundamental mechanism of viral inactivation by DUV light
The irradiation of DUV light on viruses will inhibit the replication ability of viruses, leading to the inactivation of viruses. 18–33) The DUV light refers to the wavelength range of about 200–300 nm (Fig. 3). While the inactivation effects of DUV light have been widely proven across different types of viruses, 18–33) the precise mechanism by which the DUV light inactivates viruses remains to be elucidated. 30) One possible inactivation mechanism is the molecular denaturations of nucleic acids and proteins. It is well known that the DUV light strongly interacts with nucleic acids and proteins of viruses to lead to molecular denaturations (Fig. 4). 30,53–56) The possible and predominant effect on molecular denaturation is the formation of dimers of adjacent pyrimidine (cytosine, uracil) in nucleic acids. 57,58) This denaturation of nucleic acids will inhibit viral replication because of RNA replication or protein synthesis errors using denatured nucleic acids. The other inactivation mechanisms by the deleterious effects of DUV on RNAs and proteins have also been suggested, such as the RNA-protein cross-linking and site-specific damages to RNAs and proteins. 30,57,59) Another important DUV-induced inactivation pathway is the generation of radical species, which can damage RNAs and proteins through oxidation. 60,61)
Fig. 3. (Color online) UV spectrum and its biological and chemical effects.
Download figure:
Standard image High-resolution imageFig. 4. (Color online) RNA alterations by DUV light irradiation.
Download figure:
Standard image High-resolution imageThis inactivation mechanism has not been proven in SARS-CoV-2, but the same inactivation effect can be expected even in SARS-CoV-2 due to its similar molecular components and replication mechanism compared to well-studied RNA viruses. 62,63) Actually, several studies have demonstrated the inactivation of SARS-CoV-2 by the irradiation of DUV light. 18–20,33,64–67) Furthermore, in the same manner, the viral inactivation effect can be expected for almost all viruses (including viruses having DNA as well as RNA). 31,68,69) Therefore, DUV light has the potential to be applied to various viruses, including virus variants of SARS-CoV-2, other viruses of which the inactivation effect has not been experimentally proven and novel viruses that may be emerged in the future.
3.3. Advantages of DUV-LED for viral inactivation
Viral inactivation using DUV light has several advantages comparing with the other inactivation technologies. Firstly, the operational energy consumption is low; in other words, it requires no material consumption and enables efficient energy conversion from electric power to DUV light energy. Secondly, there are no residual substances after processing because of no requirement to apply disinfectant. Thirdly, DUV light can inactivate viruses in various environments with minimal undesirable effects on the target materials. Fourthly, contact-less processing can be realized.
Various DUV light sources, such as low-pressure mercury lamp, excimer lamp and DUV-LED, have been proposed for viral inactivation. 25,34,68,70–72) Among these DUV light sources, DUV-LED is much more attractive because of its compact, lightweight and power-effective features. 34,73,74) Furthermore, DUV-LED does not use environmental pollutants such as mercury that are limited in usage in accordance with the Minamata Convention on Mercury adopted by the United Nations Environment Programme in 2013. 34,75) DUV-LED also has the designability of the operating wavelength. 73) From the above, DUV-LED would be a promising candidate for viral inactivation devices from small products for individual consumers to large products installed in buildings and facilities.
3.4. Suitable evaluation environment for the inactivation of SARS-CoV-2 by DUV-LED irradiation
For the quantitative evaluations of the inactivation profile of SARS-CoV-2 by DUV light, some precautions are required to ensure the quantitativeness of the viral inactivation effect.
The most crucial point is the optimisation of the surrounding environment of SARS-CoV-2. In general, enveloped viruses, including SARS-CoV-2, have damaged when released into air and water. 14,76–78) Therefore, SARS-CoV-2 might lose its infectivity relatively quickly. Although some viruses are infectious even in the air and water, the situation where many viruses are damaged is not favourable for the quantitative evaluation of viral inactivation. Therefore, the viruses must be stored in a culture medium and maintain their viability and infectivity. This condition must be maintained even during the DUV light irradiation. However, chemicals in the general culture medium, especially proteins and amino acids, strongly absorb DUV light. 79–81) Therefore, for the quantitative evaluation of the viral inactivation effect on SARS-CoV-2 by DUV light, it is necessary to optimise the culture medium from the viewpoints of both virus infectivity and light absorption of DUV light.
Figure 5 shows the transmittance of various culture media. 20) The culture medium (EMEM, Eagle's minimum essential media) and serum (FBS, fetal bovine serum) contain many proteins and amino acids, but phosphate buffer saline (PBS) does not. In the culture medium containing 10% serum (EMEM-10%FBS), one of the suitable viral media in terms of viral viability and infectivity, the light transmittance of 280 nm is about 7% with 3 mm optical path length, resulting in most DUV light cannot reach viruses. In contrast, in terms of light transmittance in the DUV wavelength region, the suitable media is serum-free PBS, where the light transmittance of DUV light is 90% or higher with the 3 mm optical path length. However, the SARS-CoV-2 might not exhibit sufficient viability and infectivity under the serum-free PBS. Based on the above viewpoints, the optimised culture medium in terms of viral infectivity and light transmittance is essential to quantitatively evaluate the viral inactivation effect on SARS-CoV-2 by using DUV light. An example satisfying both conditions is a culture medium obtained by diluting an EMEM containing 2% FBS with a PBS containing 2% FBS by 10-fold. 20)
Fig. 5. (Color online) Transmittance of the culture media in the DUV wavelength region (optical path length 3 mm). An example of optimised culture medium obtained by diluting an EMEM containing 2% FBS with a PBS containing 2% FBS by 10-fold is also shown. EMEM, Eagle's minimum essential medium; FBS, fetal bovine serum; PBS, phosphate buffer saline.
Download figure:
Standard image High-resolution imageThe next point to consider is the quantitativeness in evaluating the viral inactivation effect by DUV-LED irradiation. In general, DUV-LED light power density is the highest in the centre of the irradiated region and lower in the periphery. 82) The inhomogeneous irradiation power distribution of the DUV-LED might decrease the quantitativeness of evaluating the viral inactivation effect. The amount of virus suspension subjected to the evaluation is also a crucial point. As mentioned above, the culture medium of viruses strongly absorbs DUV light depending on the propagation length of DUV light even in the optimised culture medium. Thus, the thickness of the virus suspension should be thinner but enough to ensure quantitative virus experiments.
One possible apparatus to ensure the quantitativeness in evaluating the viral inactivation effect by DUV-LED irradiation is using a well plate with small liquid chambers (wells) generally used in biological experiments. Figure 6 shows an example of a DUV-LED irradiation apparatus using a well plate. 20) Since the liquid chambers of the commercially available well plates are stably manufactured, it is easy to keep the volume and thickness of the virus suspension constant in the chambers. Furthermore, the power distribution of the DUV light irradiation to the virus suspension can be relatively uniform by irradiating a sufficiently wide range against the chamber diameter.
Fig. 6. (Color online) DUV-LED irradiation system using a well plate. The virus medium-filled well plate is set just under the DUV-LED unit and is supported by the chamber alignment jigs, enabling stable irradiation of DUV-LED and quantitative inactivation effect SARS-CoV-2. (Reproduced with permission from Ref. 20.)
Download figure:
Standard image High-resolution image3.5. Inactivation effect of DUV-LED irradiation on SARS-CoV-2
The inactivation effect of DUV-LED irradiation on SARS-CoV-2 can be quantitatively evaluated by considering the viral environment and irradiation apparatus described above. Figure 7 shows the inactivation effects on SARS-CoV-2 by irradiating DUV-LED operating at the wavelength of 265, 280 or 300 nm. 20) To evaluate the actual DUV-LED energy directly imposed on the viruses, the irradiation power densities of the DUV-LEDs are recalculated based on their transmittances, which are 92 μW cm−2 for 265 nm, 83 μW cm−2 for 280 nm and 925 μW cm−2 for 300 nm. The total dose of DUV-LED energy is controlled by varying the irradiation duration. Viral infectivity of SARS-CoV-2 can be evaluated by counting the number of well-isolated plaques formed by cell shedding due to viral infection (Plaque assay). 83,84)
Fig. 7. (Color online) Inactivation of SARS-CoV-2 by using DUV-LEDs operating at the wavelength of 265, 280 or 300 nm. (a) Plaque assays for the confirmation of SARS-CoV-2 inactivation at various total doses. (b) Inactivation curves of SARS-CoV-2 against total doses of DUV-LED energy. The black broken lines show 99.9% inactivation of virus infectivity as indicated. Mean ± standard errors are shown (N = 6). (Reproduced with permission from Ref. 20.)
Download figure:
Standard image High-resolution imageThe plaque numbers decrease exponentially according to the total dose of DUV-LED energy at all wavelengths, indicating the DUV-LED operating at 265, 280 and 300 nm has the inactivation capability against SARS-CoV-2. The DUV-LED operating at 265 nm has the highest inactivation capability, followed by those at 280 and 300 nm. The total dose of DUV-LED energy required to inactivate SARS-CoV-2 by 99.9% is 1.80 mJ cm−2 at 265 nm, 3.65 mJ cm−2 at 280 nm and 23.2 mJ cm−2 at 300 nm. The irradiation energy Eirrad (mJ cm−2) is equivalent to the value obtained by multiplying the power density PLED (mW cm−2) of the DUV-LED by the irradiation duration τ (s) as follows.

Therefore, for example, in the case of the wavelength of 265 nm, if the DUV-LED with a power density of 1.80 mW cm−2 is employed, the irradiation duration of 1 s is a minimal requirement to satisfy the required dose of DUV-LED energy of 1.80 mJ cm−2 for 99.9% inactivation of SARS-CoV-2. If a DUV-LED with a higher power density can be employed, the inactivation efficiency improves proportionally to the power density of the DUV-LED. For example, the DUV-LED with a power density of 18.0 mW cm−2 could inactivate SARS-CoV-2 by 99.9% within 0.1 s. Notably, these estimated doses of DUV-LED energy for viral inactivation are the effective doses directly imposed on the viruses. Thus, if some substance, such as saliva or liquid, wrap viruses, the power density of DUV-LED should be sufficient to achieve the required effective doses directly imposed on the viruses for viral inactivation with the consideration of the substance thickness and its transmittance.
From the above, DUV-LED would be a promising candidate in inactivating the SARS-CoV-2. Although the DUV-LED operating at 265 nm has the highest inactivation capability for SARS-CoV-2, it is also necessary to comprehensively consider various factors other than the inactivation capability in the development of practical viral inactivation devices. In general, the shorter the operating wavelength of DUV-LED, the shorter the lifetime and the lower the emission power. 34,73,85) Thus, it is necessary to choose the optimum wavelength by fully considering the viral inactivation capability, lifetime and emission power of the light source for practical applications.
Notably, the irradiation dose of DUV-LED energy required for inactivating SARS-CoV-2 shown in Fig. 7 is converted into the effective doses directly imposed on the viruses with the consideration of the transmittance of the culture medium. Thus, if the surrounding environment is different, such as in air, saliva or another liquid, the power density of DUV-LED should be sufficient to achieve the required effective doses directly imposed on the viruses for viral inactivation with the consideration of the surrounding environment and its transmittance. Anyway, the quantitative evaluation method using DUV-LED provides helpful information to estimate the viral inactivation effect in a practical environment.
4. Conclusion
This paper introduced an overview of the virus inactivation technology using DUV-LED for SARS-CoV-2. The DUV-LED can provide energy-efficient and contact-less virus inactivation technology with no residual substances after treatment, little effect on targets and the applicability of various viruses, including virus variants. The DUV-LED would be a promising candidate for infectious disease control to decrease the infection risk and helps medical care, education, economy and society back to how they were before the onset of the disease.
Notably, the viral inactivation effect on SARS-CoV-2 is widely verified by many researchers; however, the sharing and standardisation of quantitative information of viral inactivation that can be used in practical use are still insufficient. The quantitative results of the viral inactivation information on not only the inactivation effects depending on operating wavelength but also the experimental environment with sufficient information for practical use must lead to the rapid and effective development of practical viral inactivation devices. Therefore, it is essential to share and standardise the viral inactivation information to prevent SARS-CoV-2 and other viruses that will emerge in the future.
Acknowledgments
We acknowledge Vice-President Kenji Kimura, Dr. Kaoru Matsuoka and Dr. Atsuo Ochi of Tokushima University for their commitment in promoting this project. We acknowledge Ms. Asaka Murakami of Tokushima University for her help in the English proofreading of the manuscript.
Biographies
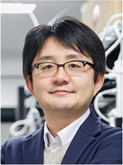
Takeo Minamikawa was born in Ibaraki, Japan, in 1983. He received the B.S., M.S., and Ph.D degrees in Engineering from Osaka University, Osaka, Japan, in 2006, 2008, and 2010, respectively. In 2011, he joined the Department of Pathology and Cell Regulation, Graduate School of Medical Science, Kyoto Prefectural University of Medicine, Kyoto, Japan. In 2015, he moved to the Institute of Technology and Science, Tokushima University, Tokushima, Japan. Since 2019, he has been an Associate Professor of the Institute of Post-LED Photonics, Tokushima University, Tokushima, Japan. His current research interests involve the interdisciplinary research of photonics and medicine, and the microscopic metrology by using vibrational spectroscopy and optical-frequency-comb.
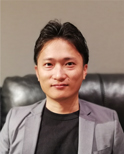
Takaaki Koma was born in Nara, Japan, in 1983. He received the B.S. degree in Health Science from Osaka University, Osaka, Japan, in 2008 and the M.S. and Ph.D degrees in Medical Sciences and Medicine from Hokkaido University, Sapporo, Japan, in 2010 and 2013, respectively. In 2013, he joined the Department of Pathology, University of Texas Medical Branch at Galveston, Texas, USA. In 2017, he moved to the Tokushima University Graduate School of Biomedical Sciences, Tokushima University, Tokushima, Japan. Since 2021, he has been an Associate Professor of the Tokushima University Graduate School of Biomedical Sciences, Tokushima, Japan. His current research focuses on the HIV-1 replication and the pathogenicity of RNA viruses such as Coronavirus and Arenavirus.
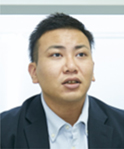
Akihiro Suzuki was born in Kyoto, Japan, in 1991. He received the B.S., M.S and Ph.D degrees in Engineering from Tokushima University, Tokushima, Japan, in 2014, 2016 and 2019, respectively. In 2019, he joined the Institute of Post-LED Photonics, Tokushima University, Tokushima, Japan. His current research interest involves the interdisciplinary research of photonics and medicine, and the biomass conversion engineering by wood decomposition and photoreaction of bacteria.
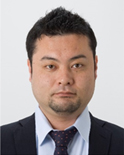
Kentaro Nagamatsu received the B.S., M.S., and Ph.D degrees in Semiconductor Engineering from Meijo University, Aichi, Japan, in 2005, 2007, and 2010, respectively. He joined Semiconductor Device Research Center, Panasonic Co. Ltd., Kyoto, Japan, in 2010. Since 2016–2019, he worked in Nagoya University, and Mie University. Since 2019 April, he joined Institute of Post-LED photonics in Tokushima University as an Associate Professor. His technology expertise includes epitaxial growth in MOVPE and HVPE, process innovation, high-power LD, UV and visible LED. He is currently with the development of light-emitting diodes in UV region.
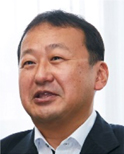
Takeshi Yasui received the first Ph.D degree in Engineering from the University of Tokushima, Tokushima, Japan, in 1997, and the second Ph.D degree in Medical Science from Nara Medical University, Nara, Japan, in 2013. From 1997 to 1999, he worked as a Post-Doctoral Research Fellow in the National Research Laboratory of Metrology, Japan. He was with the Graduate School of Engineering Science, Osaka University from 1999 to 2010, and was briefly with the University of Bordeaux I in 2007 and 2012, and with the University of Littoral Côted'Opale in 2010 as an Invited Professor. He is currently the Director and a Professor of the Institute of Post-LED Photonics, and a Vice Director of Research Organization, Tokushima University. He is also an Invited Professor of the Graduate School of Engineering Science, Osaka University. His research interests include THz instrumentation and metrology, second-harmonic-generation microscopy, and optical frequency comb.
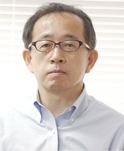
Koji Yasutomo is a Professor of Graduate School of Biomedical Sciences, an Adjunct Professor of Institute of Advanced Medical Sciences, a Vice Director of Institute of post-LED photonics at Tokushima University. He received his M.D. and Ph.D at Tokushima University and did postdoctoral works in NIH, USA. His research group is focused on T cell biology and genetics of autoinflammatory disorders. He is the recipient of Japan Society for Immunology Prize and Kawano Prize in Pediatric Medicine.
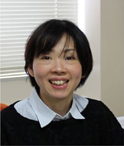
Masako Nomaguchi is a Professor for Department of Microbiology of Graduate School of Biomedical Sciences, Tokushima University. She received her Ph.D from University of Occupational and Environmental Health, Fukuoka, Japan. She has been working on HIV/SIV research, especially focusing on virus mutation and adaptation. Recently, she and her collaborators have started basic and clinical studies on SARS-CoV-2, including development of anti-viral drugs and virus inactivation by DUV-LED.