Abstract
The well-defined Lutetium (III) hexacyanoferrate microparticles were electrodeposited on poly(taurine) modified glassy carbon electrode (GCE), namely (LuHCF/poly(taurine)-GCE). The as-fabricated LuHCF/poly(taurine)-GCE possesses excellent electrochemical activities and high sensitivity toward the sensing of sulfite. The electrochemical oxidation of sulfite on LuHCF/poly(taurine)-GCE is studied by using Cyclic Voltammetry (CV), and Differential Pulse Voltammetry techniques (DPV). Remarkably, the reported modified electrode exhibits high sensitivity (448 μA mM−1 cm−2) and limit of detection (1.33 μM) toward the electrochemical oxidation of sulfite with superior selectivity and practicability.
Export citation and abstract BibTeX RIS

This is an open access article distributed under the terms of the Creative Commons Attribution Non-Commercial No Derivatives 4.0 License (CC BY-NC-ND, http://creativecommons.org/licenses/by-nc-nd/4.0/), which permits non-commercial reuse, distribution, and reproduction in any medium, provided the original work is not changed in any way and is properly cited. For permission for commercial reuse, please email: oa@electrochem.org.
Sulfite is extensively used as an antioxidant, food additive, bacterial growth, and regulates the enzymatic and non-enzymatic browning response during the protection and food storage. 1–3 Moreover, it acts as a reducing agent, which is used in boiler and boiler feed waters for regulating the level of dissolved oxygen. Nevertheless, the large quantity of the sulfite in boiler water can cause the corrosion. 4 Although sulfite was used in wide applications, the usage of sulfite should be limited since it causes serious side effects to human health. Because, the presence of excess amount of sulfite in water and other food items is an origin to many major problems such as asthmatic, allergic reaction, skin allergy, gastrointestinal disease and breathing difficulties etc.,. 5 Besides, a micro level content of sulfites in food samples also leads to unconsciousness, permanent brain damage and sometimes death. Hence, it is highly important to control and determine the concentration of sulfite in foods and other industrial areas. For many years, the numerous techniques such as luminol chemiluminescence, 6 flow amperometry technique, 7 flow injection analysis, 8 colorimetric technique, 9 fluorescence technique, 10 ratiometric fluorescent technique, 11 chromo fluorogenic, 12 capillary electrophoretic, 13 spectrophotometric method, 14 rapid micros-till, 15 zone electrophoresis, 16 HPLC 17 and electrochemical technique 18 have been used for the sulfite detection. Compared to other methods, the electrochemical techniques have many advantages such as low-cost, less time-consuming process and simple instrumental setup for the sulfite determination.
On the other hand, a diverse of materials have been used for sensing of sulfites such as carbon nanomaterials, metal oxides, 19 metal nanoparticles 20 and metal hexacyanoferrate (MHCF). 21 Among them, MHCF is a stable and inorganic poly-nuclear compound with a zeolite structure and fcc lattice. In recent years, a variety of metal hexacyanoferrate has been synthesized through the electrochemical deposition with different morphologies. So far, star-like terbium hexacyanoferrate 22 and flower like samarium hexacyanoferrate, 23 flower and Christmas tree like cerium hexacyanoferrate, 24 yttrium (III) hexacyanoferrate micro flowers 25 and switching morphologies of praseodymium hexacyanoferrate 18 have been reported for sensor applications. Notably, the previously reported MHCFs particles were prepared via electrochemical deposition technique owing to its simple cost, low time consuming and well-defined structure forming ability by controlling the time, cycle number and nature of the supporting electrolytes.
In order to extend the application of MHCF in the field of the electrochemical sensor, we have electrochemically deposited the Lutetium (III) hexacyanoferrate (LuHCF) on poly(taurine) modified GCE and further used as a working electrode for the electrochemical oxidation of sulfite as shown in Scheme 1. In general, poly(taurine) is an amino acid with electron-rich N atoms, 26 which has been made as the composite materials with carbon, metal oxides and metal nanoparticles for several sensor applications, e.g, poly(taurine)/TiO2-Graphene for determination of acetaminophen and caffeine, 27 Gold and platinum modified poly(taurine) was used for hydrazine sensor. 28 Relative to our previous report B. Devadas et al., 18 the present modified electrode LuHCF/ poly(taurine)/GCE exhibited the low limit of detection and high sensitivity respectively. Moreover, poly(taurine) contains sulfonic groups and electron rich N atoms, it ensures the electrical conductivity and electrochemical stability 29 of LuHCF/(poly(taurine) toward electrochemical sensor relative to our previous report PrHCF/GCE. 18 Thus, we believe that the synergistic effect of poly(taurine) and LuHCF would be more suitable for the sensitive detection of sulfite.
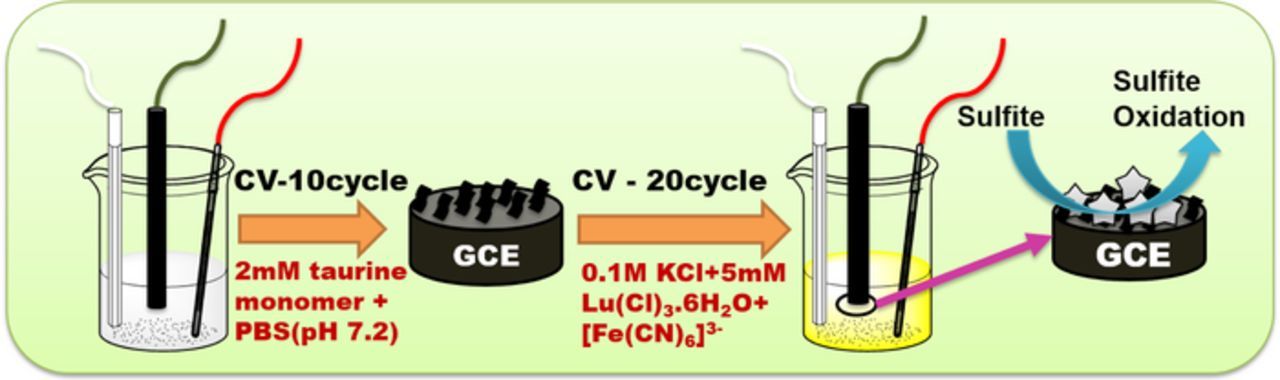
Scheme 1. Electrochemical synthesis of LuHCF/Poly(taurine)-GCE for simultaneous detection of sulfite.
Experimental
Materials and methods
Lutetium (lll) chloride hexahydrate (LuCl3.6H2O), sodium hydroxide (NaOH), potassium nitrate (KNO3) and sodium nitrate (NaNO3) were purchased from Sigma Aldrich. Potassium ferricyanide, lithium nitrate (LiNO3) and Sodium sulfite were purchased from Wako pure chemical industries Ltd. All the chemicals were of analytical grade and used as received. Besides, the experimental solutions were prepared using double distilled deionized (DI) water.
Preparation of poly(taurine) modified glassy carbon electrode
A simple electro-polymerization has been followed to prepare poly(taurine) modified glassy carbon electrode (GCE). Prior to the experiment, GCE was well polished by using alumina slurry followed by washing with ethanol and double distilled water (DD). Then, the polished GCE was immersed in 10 mL of phosphate buffer solution (PBS pH 7) containing 2 mM of taurine monomer. The electropolymerization was performed in CV by applying the set of potential from 2.0 to −1.5 V at a scan rate of 50 mV/s for 10 deposition cycles. After the electropolymerization, the modified electrode was rinsed with DD water to remove loosely adsorbed monomer.
Electrochemical preparation of LuHCF/ploy (taurine) modified GCE
The viable electrochemical deposition was performed as follows; The poly(taurine) modified GCE was placed in 0.1 M KCl solution containing 5 mM of Lutetium (lll) chloride hexahydrate and K3Fe(CN)6 and followed the consecutive 20 CV cycles deposition in the potential range from 0.8 to −0.2 V at the scan rate of 50 mV/s. In result of this experiment, the star-like structure of LuHCF was successfully deposited on poly(taurine) modified GCE. Finally, the LuHCF/poly(taurine) modified GCE was washed again with DI water to remove the loosely adsorbed LuHCF particles and dried at room temperature. The same procedure was followed to prepare the LuHCF/GCE without poly(taurine).
Characterization techniques
The surface morphological studies were carried out using a Hitachi S-3000H scanning electron microscope (SEM) with affiliated energy dispersive X-ray spectroscopy (EDX) using HORIBA EMAX X-ACT. The X-ray diffraction pattern was recorded using a Rigaku, MiniFlex II instrument. The CHI611A workstation with conventional three electrodes electrochemical cell setup was used for the electrochemical synthesis and characterization. In this regard, GCE employed as a working electrode, Ag/AgCl as a reference electrode and platinum wire as a counter electrode. The Electrochemical impedance spectroscopy (EIS) was performed by using ZAHNER impedance analyzer.
Results and Discussion
Mechanism in the preparation of LuHCF/poly(taurine) modified GCE
The electropolymerization of the poly(taurine) was performed by our previously reported article. 30 The primary set of potential range from + 2.0 and −1.5 V has been fixed for the electropolymerization and their corresponding CV profile is displayed in Fig. 1A. It can be seen that one obvious reduction peak and two oxidation peaks for electropolymerization reaction. The reduction peak indicates the reduction of taurine monomer and the two oxidation peaks correspond to polymerization film the deposition on GCE surface. At the end of the 10th cycle, the peaks were attained to saturation condition and poly(taurine) film was completely deposited on GCE surface. The as-prepared poly(taurine)/GCE was further modified by LuHCF using similar electrodeposition method. The CV profile for the electrochemical deposition of LuHCF as shown in Fig. 1B, which shows the pair of peak validates to the reaction of Fe (CN)6 3−/4−, those peaks were decreased with the increase of CV scans. From the 2nd scan, the pair peaks were observed which increased depends on the CV scan and specifies that the deposition of LuHCF film on poly(taurine)/GCE. The formation of LuHCF complex can be expressed as following reaction mechanisms,
![Equation ([1])](https://content.cld.iop.org/journals/1945-7111/165/10/B469/revision1/d0001.gif)
![Equation ([2])](https://content.cld.iop.org/journals/1945-7111/165/10/B469/revision1/d0002.gif)
Figure 1. (A) CV curve for the electrodeposition of poly(taurine) in monomer (2 mM) containing 0.05 M PBS (pH 7.2) at scan rate of 50 mV s−1. (B) CV profile for electrodeposition of LuHCF in 0.1 M KCl containing 5 mM LuCl3.6H2O and K3Fe(CN)6 3− at scan rate of 50 mV s−1.
In order to form the uniform LuHCF deposition, the equal molar ratio (5 mM) of metal precursor (Lu) and ferricyanide has to be maintained.
Characterization of LuHCF/poly(taurine)
Fig. 2 shows the SEM image of LuHCF on poly(taurine) film. As shown in the figure, the star-like structure of LuHCF was clearly observed with the uniform size from 3 to 4 μm (Fig. 2B). In addition, the electrodeposited LuHCF was confirmed by energy dispersive X-ray (EDX) spectroscopy. The obtained result can be seen in Fig S1, which shows the major peaks for the corresponding elements of Lu, K and Fe, which confirms the presence of major constituent elements in prepared LuHCF film. And also, the quantitative atomic percentage of Lu, Fe and K were calculated to be 63.2%, 21.6, and 15.2% respectively. This result confirmed the formation of LuHCF complex on poly(taurine) modified film. Moreover, we studied the effect of supporting electrolyte on the morphology of LuHCF. Fig. 3 depicts the morphological differences of electrodeposited LuHCF in presence of different cations (K+, Na+, Li+ and H+) containing the electrolyte. Among them, Li+ and H+ containing electrolyte shown the undefined structure of LuHCF. Besides, the electrodeposited LuHCF was not in uniform size and deposited randomly. In contrast, K+ and Na+ containing electrolytes shown the respective morphological effect on electrodeposited LuHCF, whereas the obtained particles having a well-defined structure with uniform size. Monovalent cation mainly affects the morphology, here increment of affinity and hydrated ionic radius leads to uniform morphology (K+ >Na+ >Li+ >H+). 18 In addition, the electrochemically active surface area of LuHCF samples that were electrochemically deposited from solutions containing different supporting electrolytes (salts or acids) including KCl, NaCl, and LiCl, and HCl. As shown in Figs. 4A, 4B, the electrochemical active surface areas determined using Fe(CN)6 4− solution as a redox probe from the Randel Sevick equation shown in Equation 1 follows the order of K+ >Na+ >Li+ >H+ and was determined to be 0.12, 0.11, 0.09, 0.08 and 0.069 cm2 for KCl, NaCl, LiCl, HCl and bare GCE, respectively. 31
![Equation ([3])](https://content.cld.iop.org/journals/1945-7111/165/10/B469/revision1/d0003.gif)
Figure 2. (A, B) SEM images of LuHCF obtained for 20 cycles deposition at different magnification.
Figure 3. SEM image of LuHCF at different electrolyte (A) KCL, (B) NaCl, (C) LiCl, and (D) HCl.
Figure 4. (A) CV curves for different electrolyte deposition of LuHCF modified electrodes were calculated the active surface area in 0.1 M KCl and 5 mM of [K3Fe(CN)6]3−/4− electrolyte. (B) The bar diagram of different modified electrodes was calculated the active surface area. (C) The K+ containing electrolyte deposition of LuHCF electrode for the different scan rate variation in 0.1 M KCl and 5 mM of [K3Fe(CN)6]3−/4− electrolyte and the corresponding linear calibration plots (D).
Where n is the number of electrons involved in the redox reaction (n = 1), A is the area of the electrode (cm2), D is the diffusion coefficient of the ferricyanide (cm2 s −1), C is the concentration of the ferricyanide in the bulk solution (mol cm−3), and γ is the scan rate (V s−1).
Fig. 4C as shown, the CV profile of modified electrode was exhibited the different scan rate 10 to 100 mV/s in 5 mM [Fe(CN)6]3/4− containing 0.1 KCl electrolyte and the liner redox peak current increase with increasing scan rate. Further, the linear relationship between the increase in the redox peaks current vs. square root of scan rates were determined by the linear calibration plot as shown in Fig. 4D. The linear regression equation was calculated value of Ipa (μM) = 13.19(V1/2 (mVs−1)1/2 + 1.55; R2 = 0.9995 and Ipc (μA) = −11.443(V1/2 (mVs−1)1/2 + 14.147; R2 = 0.9969. It has to exhibit the all electrochemical redox peak process in ferricyanide solution and the active electrode surface area also determinate. Therefore, the K+ containing electrolyte has been chosen as an optimized condition for the electrodeposition of LuHCF.
The structure and phase of electrodeposited LuHCF on poly(taurine) was analyzed using the X-ray diffraction technique. As shown in the Fig S2, the XRD of LuHCF complex shows the major diffraction peaks 15°, 19° and, 25° with high intensity. The indicated 2θ values were well matched with the previously reported lanthanide metal hexacyanoferrate. 30 According to these number, the electrodeposited LuHCF has ortan horhombic structure model and Cmcm space group. This result validates that the electrodeposited LuHCF complies with lanthanide group (rare earth metal hexacyanoferrate) complex.
Electrochemical characterization of LuHCF/poly(taurine)-GCE
Electrochemical impedance spectroscopy (EIS) is an excellent electrochemical technique to analyze the physical properties of an active material. 32 It provides the reasonable value of lower charge transfer resistance (Rct ), which relatively depends upon the electron transfer reaction between the electrolyte and surface of the electrode. In general, the radius of the semicircle of EIS curve is directly proportional to the Rct of the modified GCE. Fig. 5 shows the Nyquist plot of LuHCF/poly(taurine)-GCE and bare GCE analyzed in 0.1 M KCl solution containing 5 mM of [K3Fe(CN)6]3−/4− as the supporting electrolyte. The corresponding electrical equivalent Randles circuit shown in the inset of Fig. 5 which consist of solution resistance (Rs ), charge transfer resistance (Rct ), Warburg impedance (W) and double layer capacity (Cdl). 33,34 The calculated Rct values were 77.49 Ω and 1087.0 Ω, 125.47, 475.2 Ω for LuHCF/poly(taurine)-GCE and LuHCF/GCE, poly(taurine)/GCE, and bare GCE respectively. This result reveals that the proposed LuHCF/poly(taurine)-GCE exhibited an ultra-low charge transfer resistance with respect to the small semicircle appears than that of LuHCF/GCE, poly(taurine)/GCE, and bare GCE as shown in Fig. 5. Poly(taurine) has high electron rich N atoms and sulfonic functional group so it plays the main role in order to reduce the charge transfer resistance of GCE and LuHCF/GCE electrodes. Therefore, the electro-deposition of poly(taurine) is an excellent way to further enhance the electron transfer ability of LuHCF/GCE.
Figure 5. EIS spectra of Bare GCE, LuCHF/GCE, poly(taurine)/GCE, and LuHCF/poly(taurine)/GCE in 0.1 M KCl and 5 mM of [K3Fe(CN)6]3−/4−.
Fig. 6A shows the CV profile of LuHCF/poly(taurine)-GCE, poly(taurine)/GCE, LuHCF/GCE and bare GCE electrodes with and without sulfite (740 μM) in N2 saturated 0.1 M KCl at the scan rate of 50 mV s−1. It is clear that the LuHCF/poly(taurine)-GCE shows the excellent oxidation current response (Ipa = 7.3 V) for the addition of sulfite (740 μM) at an oxidation potential of Epa = 0.65 V. The recorded oxidation current of LuHCF/poly(taurine)-GCE was higher than other modified electrodes such as poly(taurine)/GCE, LuHCF/GCE and bare GCE. Hence, we concluded that the synergistic effect between LuHCF and poly(taurine) is found as a good way for selective sensing of sulfite even at a very low concentration. This excellent electrocatalytic activity may due to the interaction between the functional groups of poly(taurine) and active metal center of LuHCF complex. Fig. 6B shows the CV curve for LuHCF/poly(taurine)-GCE by varying the scan rate from 10 to 100 mV s−1 in N2 saturated 0.1 M KCl. The well-defined electrochemical reversible behavior was observed for LuHCF/poly(taurine)-GCE with increasing the scan rate. The corresponding linear calibration plot was drawn for the scan rate (ʋ1/2 ) vs current, it provides the linear regression equation with calculated R2 as shown in the inset of Fig. 6B. The result implies that the overall process of LuHCF/poly(taurine)-GCE modified electrode was controlled by the surface-controlled process.
Figure 6. (A) LuHCF/poly(taurine)/GCE (a), poly(taurine)/GCE (b), LuHCF/GCE (c), and Bare GCE (d)with sulfite 740 μM concentration in N2 saturated 0.1 M KCl at the scan rate of 50 mV s−1. (B) LuHCF/polymer/GCE different scan rate (inset: Linear calibration plot with square root vs mVs−1).
DPV studies at LuHCF/poly(taurine)-GCE for the electrochemical oxidation of sulfite
The prepared LuHCF/poly(taurine)-GCE was analyzed in the DPV technique to calculate its electrocatalytic properties related to essential electrochemical parameters such as sensitivity, limit of detection and linear range. Fig. 7 shows the relative DPV curve of LuHCF/poly(taurine)-GCE for increasing the linear concentration of sulfite in N2 saturated 0.1 M KCl electrolyte. The increasing oxidation current was observed by increasing the concentration of sulfite. The inset of Fig. 7 shows the linear calibration plot with corresponding linear regression equation including calculated R2 = 0.9981. The high active surface area has given superior performance with maximum signal to noise ratio (SNR), the obtained limit of detection and sensitivity is about 1.33 μM (SNR = 3) and 0.448 μA μM−1 cm−2, respectively. The obtained results were compared with previously reported literature which displayed in Table S1. Hence, it is conclusive that the reported LuHCF/poly(taurine)/GCE exhibited substantial electrocatalytic sensing behavior toward the electrochemical sensing of sulfite.
Figure 7. DPV studies of different concentration of sulfite in N2 saturated 0.1 M KCl (Inset: Linear calibration with sulfite concentration vs current).
Interference studies
The selectivity of the sensor is highly important for the practicability. Thus, the selectivity of LuHCF/poly(taurine)-GCE toward the detection of sulfite was recorded by using DPV technique under the same experimental condition as followed in Fig. 8. As the result, Fig. 8A shows the DPV curve for the addition of sulfite (1 mM) and 100 fold higher concentration of interference species such as sulfide, sulfate, nitrite, nitrate, ascorbic acid, sucrose, glucose, tartaric acid, and citric acid. The corresponding relative error bar diagram is given in Fig. 8B. It suggesting that the presence of interference species causes the negligible amount of signal changes (less than 5%) only, which proposed that the LuHCF/poly(taurine)-GCE exhibits the excellent selectivity for electrochemical detection of sulfite.
Figure 8. (A) DPV curve for interference study, and (B) Corresponding relative error percentage bar diagram.
Stability
The stability studies were important for practical application of sensor, in this row stable electrochemical performance (stability) is also necessary. Fig. S3 (A) shown, the DPV curve of LuHCF/poly(taurine)-GCE were carried out the presence of a sulfite (100 μL) in 0.1 M KCl electrolyte at a scan rate of 50 mV s−1 and the modified GCE was performed very well up to 11 days. Further, an examination was carried out every day after that electrode stored at room temperature. Poly(taurine) has helped to stabilize the electrochemical activity of LuHCF, in Fig. S3 (B) up to 6 days no change in DPV current response after that in considerable changes happened. Finally, stability study has revealed LuHCF/poly(taurine) modified electrode has good stability for a long time.
Real sample analysis
To evaluate the practical application of LuHCF/poly(taurine)-GCE, which was analyzed to detect sulfite from tap water and the purified drinking water from the nearby supermarket sample. Prior to analysis, 10 mg per ml of the sulfite was dispersed in tap and drinking water (10 ml) through sonication for 15 minutes. The LuHCF/poly(taurine)-GCE was tested for detection of sulfite for the tap water sample using the standard addition method as shown in Fig. S4. In addition, the recovery values of sulfite in tap and drinking water samples were calculated by using formula (R = (Added/found)*100) and the average recovery values were exhibited in the 98.95% and 95.77% respectively. The LuHCF/poly(taurine)-GCE was observed at well-defined oxidation of sulfite from the tap water and the obtained results were suitable for real-time application. There are no other peaks observed for the mediated oxidation of tap water, it might be due to the better electrochemical performance of the reported sensor.
Conclusions
In conclusion, a facile electrochemical method was achieved for the fabrication of LuHCF/poly(taurine)-GCE for the detection of sulfite. The morphologies of LuHCF particles with respect to the effect of electrochemical techniques, electrolytes, and deposition cycles were investigated. The modified electrode was observed with high sensitivity and limit of detection and also with a well-defined oxidation of sulfite in real sample analysis. Hence, we believe that the reported modified electrode would reach the prototype level applications.
Acknowledgments
This work was financially supported by the Ministry of Science and Technology, Taiwan (MOST 106-2113-M-027-003 to SMC). The support offered by the National Taipei University of Technology for carrying out this work is gratefully acknowledged.
ORCID
Shen-Ming Chen 0000-0002-0132-9161