Abstract
The effect of vinylene carbonate (VC) as an electrolyte additive on the electrochemical performance of Si film anode is reported in this paper. The cycle performance and efficiency of Si film anode were enhanced significantly with the presence of VC in electrolyte. It was found that the SEI layer formed in VC-containing electrolyte was impermeable to electrolyte and its impedance remained almost invariant upon cycling. Moreover, silicon oxide was formed in solid electrolyte interface layer of Si film anode due to the reaction of lithiated silicon with permeated electrolyte in both VC-free and VC-containing electrolytes.
Export citation and abstract BibTeX RIS
To meet the increasing demand for lithium-ion batteries with high energy density due to the rapid development of portable electric devices and (hybrid) electrical vehicles, some new anode materials with high capacity and long cycle performance have been studied extensively.1–4 Among them, Si has attracted much attention because it has the highest specific capacity for any of anode materials studied to date.5 But, Si undergoes a dramatic volume change during
insertion and extraction. This causes the pulverization of Si particles and loose contacts between silicon particles and current collector, resulting in the mechanical instability and poor cyclability. Thus, amorphous Si film has been considered as a promising anode material for lithium-ion batteries because of its large capacity and excellent cycle performance. Currently, Si film anodes prepared by various deposition methods including chemical vapor deposition (CVD), sputtering, and evaporation have been developed by many research groups.6–11 The electrochemical performance of Si film anode is decided by many factors, such as thickness of film, roughness of substrate, and parameters of deposition. However, the effect of electrolyte on electrochemical performance of Si film anode has not yet been reported. For graphite anode, it has been reported that electrolyte additive played an important role in improving the electrochemical properties. A slight presence of electrolyte additive can result in a critical difference in electrochemical behavior of graphite anode, as evidenced by the well-known example of vinylene carbonte (VC), vinyl ethylene carbonate, vinyl acetate, and so on.12–14
In this paper, we first studied the effect of VC electrolyte additive on the electrochemical performance of Si film anode prepared by ion beam sputtering deposition. The presence of VC in electrolyte improved the cycle performance of Si film anode greatly.
Experimental
Si films were prepared by ion beam sputtering deposition from an n-type Si target on thick Cu foils (
diam) as current collector. The working pressure was
in Ar atmosphere, and the sputtering angle was
. The surface roughness of the Cu foils
(rms). The thickness of Si film was
.
The 2025-type half-cells containing testing electrode, separator, electrolyte, and lithium foil as counter electrode were assembled in a glove box filled with pure Ar. The electrolytes used in the experiments were ethylene carbonate/dimethyl carbonate (EC:DMC) (1:1 in volume) with or without
VC additive. Cycle performance was carried out at a constant current density of
using Land battery test system with the cutoff potentials being
vs
for discharge and
vs
for charge. After different cycles, electrochemical impedance spectroscopy (EIS) measurements were performed using electrochemical workstation (Shanghai Chenhua Instrument Co., China). The impedance spectra were measured after the cell had been discharged to
and the voltages were in equilibrium.
After electrochemical testing, the Si film anode in the state of deinsertion was removed from the coin cell and rinsed with highly purified DMC to remove electrolyte. The surface morphologies of Si film before and after the first cycle were observed by scanning electron microscopy (S-3000N, Hitachi, Japan) equipped with an energy-dispersive X-ray (EDX) detector. The organic components of the SEI layers were characterized by a Fourier transform infrared (FTIR) spectrometer (Nexus 470, Nicolet, USA). X-ray photoelectron spectroscopy (XPS) experiments were carried out on a PHI-5000C ESCA system (Perkin Elmer, USA) with
radiation
.
Results and Discussion
Figure 1 shows the cycle performance and efficiency of Si film anode in VC-containing and VC-free electrolytes. Si films tested both in VC-containing and VC-free electrolytes exhibit a first discharge capacity of 2777 and , respectively, and the initial coulombic efficiency of 72.5 and 61.1%, respectively. Both samples show a reversible capacity of about
. In VC-containing electrolyte, Si film exhibits superior electrochemical performance. The capacity is kept stable even up to 200 cycles. The efficiency increases to
after 10 cycles, and remains stable in subsequent cycles. In contrast, in VC-free electrolyte, severe capacity fade occurs. The capacity drops to
after 200 cycles. Furthermore, the efficiency can only increase to 96%, and fluctuates during cycling. The difference of cycle performance and efficiency of Si film anode in VC-containing and VC-free electrolytes may be attributed to the different properties of SEI layer formed in initial several cycles.
Figure 1. Comparison of cycle performance and efficiency of Si film anode in VC-containing and VC-free electrolytes.
Scanning electron microscopy (SEM) images of Si film anodes before and after cycling are shown in Fig. 2 to compare the morphologies of SEI layers formed in different electrolytes. Figure 2a shows that the surface of Si film is clean before cycling, although it is rough due to the surface roughness of Cu foil substrate. When the Si film electrode is discharged to , the surface is covered with the SEI layer which is the reduction product of electrolyte including solvent and salt anion. The SEI layer formed in VC-free electrolyte is nonhomogeneous and embeds with some spherical particles with about
in size, as shown in Fig. 2b. EDX study is carried out on such particles. The compositions of the particle and the flat spot on the SEI layer (as shown in the direction of arrow) are presented in Table I. The content of F in the particle is much higher than in the flat spot. Andersson15 had also shown the similar SEI morphology on the surface of graphite anode after three cycles and elevated temperature storage. He verified that the crystallites were
crystals, and he thought that the isolated
crystals that were permeable to electrolyte caused the degradation of graphite electrode. The SEM image of the SEI layer formed in VC-containing electrolyte shows a smooth and uniform morphology, as seen in Fig. 2c. No spherical crystallite appears on the surface of SEI layer. However, the SEI layer displays a series of cracks in the valleys between the hills. We think that the cracks are caused by the shrinkage of Si film during lithium ions extraction. When the lithium ions are extracted from Si electrode, most of the contraction of Si layer must be in the vertical direction to the substrate. SEI layer in the valleys cannot bear the contraction stress of the two sides of walls of the valley, resulting in the cracks. It is concluded that the presence of VC in electrolyte results in very different surface morphology of the SEI layer and configuration of
, which may be the key factors to the electrochemical performance of Si film.
Figure 2. SEM images of Si film anodes before and after cycling in different electrolytes. (a) Before cycling. (b) After the first cycle in VC-free electrolyte. (c) After the first cycle in VC-containing electrolyte.
Table I. The compositions of the particle and the flat area on the SEI layer estimated from the EDX data.
Elements | Flat spot | Particle |
---|---|---|
C (%) | 36.60 | 14.95 |
O (%) | 58.09 | 60.08 |
F (%) | 5.31 | 24.00 |
P (%) | 0 | 0.97 |
Figure 3 shows the changes of electrochemical impedance spectra of Si film anode upon cycling in VC-free and VC-containing electrolytes. As already discussed in detail,16 the high-frequency semicircle relates to resistance for migration through SEI layer, and the medium-frequency semicircle relates to charge transfer resistance between SEI layer and electrode interface, and the low frequency is attributed to Warburg impedance (diffusion of
in the electrode) and insertion capacitance (accumulation of
in the electrode). As seen in Fig. 3a, the diameters of high frequency semicircles increase dramatically with the increasing of cycling times in VC-free electrolyte. The increasing of SEI layer impedance may be attributed to the increasing of SEI layer thickness. As shown above, isolated LiF crystals are formed in the SEI layer of Si film in VC-free electrolyte. The electrolyte can permeate the SEI layer during cycling. Therefore, a new SEI layer is formed constantly on the surface of Si film in each cycle, resulting in the increasing of thickness of SEI layer. This then causes the increasing of anode polarization, which degrades the electrochemical performance of Si film. Figure 3b shows the pronounced impact of VC on the impedance behavior of electrodes. Both the overall SEI layer impedance and its fluctuations upon cycling are smaller in VC-containing electrolyte, and demonstrates that impermeable SEI layer is formed on the surface of Si film, and it remains stable upon cycling.
Figure 3. Nyquist plots for changes of electrochemical impedance spectra of Si film anode upon cycling in VC-free (a) and VC-containing (b) electrolytes.
To obtain information on the chemical component of the SEI layer, FTIR and XPS spectra of the SEI layers formed in two kinds of electrolytes were measured. Figure 4 shows the comparison of FTIR spectra of SEI layers formed in VC-containing and VC-free electrolytes. The pronounced peaks at ,
,
,
, and
are attributed to
, which are the major reduction products of EC.17 Another two peaks at 1162 and
may relate to polycarbonates that are formed by some polymerization of EC and DMC.12 The peaks around 1500–1420 and
should be attributed to
.17 Spectrum related to the SEI layer formed in VC-containing electrolyte contains not only the typical above-mentioned peaks of
, polycarbonate and
, but also a poly(VC) peak at
18 that is absent in spectrum of SEI layer formed in VC-free electrolyte. The spectral differences of the SEI layers described above are attributed to the possible polymerization of VC and/or the VC reduction products via their double bond. The reduction mechanisms of VC were suggested in the literature.18
Figure 4. Comparison of FTIR spectra of SEI layers formed on Si film anode in VC-containing and VC-free electrolytes.
A semiquantitative analysis of the XPS spectra is carried out based on atom sensitivity factor. The atomic concentration of various elements can be calculated according to
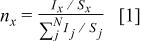
where is the peak area of the element,
is the atom sensitivity factor, and
is the total of elements. The comparison of atomic concentration of various elements in SEI layers formed in VC-free and VC-containing electrolytes is shown in Fig. 5. The atom concentration of F in SEI layer formed in VC-containing electrolyte is obviously lower than that in VC-free electrolyte. The possible components containing F are
and
in the SEI layer. Because the content of P that derived from
is very low, it indicates that the content of
decreases greatly in the SEI layer formed in VC-containing electrolyte.
Figure 5. Comparison of atomic concentration of various elements in SEI layers formed in VC-free and VC-containing electrolytes.
Note that the silicon oxide appears in SEI layers formed in both VC-free and VC-containing electrolytes. Figure 6 shows the XPS spectra. Because the concentration of silicon oxide is very low in the top surface of SEI layers, the intensity of the spectra is weak and the shape is irregular due to the accuracy of XPS instrument. The peaks located at
can be assigned to
. The formation mechanism of
in the SEI layer on Si anode has not been reported in the literature. We think that the mechanism may be very similar to the formation mechanism of
on Sn film electrode suggested by Shieh,19 but the formation process is different. The possible reaction can be interpreted as follows

Figure 6.
XPS spectra of SEI layer formed in VC-free and VC-containing electrolytes.
It is likely that the formation of silicon oxide is undergone mainly in the insertion process. In the first
insertion process, the initially formed SEI layer is cracked due to the expansion of silicon. As a result, the electrolyte permeates through the damaged SEI layer and reacts with lithiated silicon to form silicon oxide. In the
deinsertion process, the SEI layer is broken in the valleys as described in the SEM, and silicon oxide can also be formed in the cracks.
Conclusion
In this paper, the effect of VC as electrolyte additive on Si film anode was studied in detail. The cycle performance and efficiency of Si film anode were enhanced significantly with the presence of VC in electrolyte. The reversible capacity remained stable even up to 200 cycles. The superior electrochemical performance of Si film in VC-containing electrolyte was attributed to the advanced properties of SEI layer formed in initial several cycles. The SEI layer formed in VC-containing electrolyte showed smooth and uniform morphology. Its impedance remained almost invariant upon cycling because the SEI layer was impermeable to electrolyte and remained stable. However, in VC-free electrolyte, the impedance of SEI layer increased constantly upon cycling due to the increasing of SEI layer thickness, and this caused the increasing of anode polarization, which resulted in the degradation of electrochemical performance of Si film anode. It was also found that silicon oxide was formed in SEI layer of Si film anode due to the reaction of lithiated silicon with permeated electrolyte in the insertion and deinsertion processes.
Acknowledgments
This study was supported by National High-Tech Research and Development Program of China.
The Chinese Academy of Sciences assisted in meeting the publication costs of this article.