Abstract
The field of dentistry is consistently innovating with the introduction of novel hybrid and polymer materials for computer-aided design and manufacturing (CAD/CAM). It is noteworthy that the temperature within the oral cavity has a significant impact on the strength of new biomaterials utilized for CAD/CAM fabrication of fixed partial dentures (FPDs). Studies have demonstrated that alterations in intraoral temperature may significantly affect the longevity and durability of dental restorative materials. This study aimed to evaluate the flexural strength, flexural modulus, and effect of thermal aging on CAD/CAM restorative materials. Five CAD/CAM materials were investigated: nano-ceramic-hybrid (GR), polymer-infiltrated-ceramic-network (VE), polyether-ether-ketone (PK), fiberglass-reinforced epoxy-resin (CT), and Feldspar Ceramic (VB). A total of 100 bar-shaped specimens were prepared (N = 20). Each group was subdivided into thermocycling (TC) and no-thermocycling (NTC) subgroups (n = 10). All the specimens underwent a 3-point bending test. The mean flexural strengths and moduli were statistically analyzed using paired t-test, analysis of variance (ANOVA), and Bonferroni pair-wise comparison (p < 0.05). Significant differences were observed in the flexural strength (FS) and modulus (E) between the materials (p < 0.001). GR had the highest FS among tested hybrid materials. NTC CT had the highest FS (924.88 ± 120.1 MPa), followed by GR (385.13 ± 90.73 MPa), then PK (309.56 ± 46.84 MPa). The FS of brittle ceramic VB was the lowest (p < 0.001), but similar to that of PICN VE. Only resin-containing VE and CT significantly decreased in E after thermocycling (p < 0.01, p = 0.013), showing the softening effect of thermocycling on their resin matrix. It can be concluded that new hybrid materials (GR) had higher flexural strength than feldspar ceramic and other resin/polymeric CAD/CAM materials. Polymeric PEEK and GR hybrid materials were resistant to significant deleterious effects of TC. Therefore, they would be appropriate for situations with a higher stress load.
Export citation and abstract BibTeX RIS

Original content from this work may be used under the terms of the Creative Commons Attribution 4.0 licence. Any further distribution of this work must maintain attribution to the author(s) and the title of the work, journal citation and DOI.
1. Introduction
Computer-aided design/computer-aided manufacturing (CAD/CAM) technology's use to mill dental restorations has grown exponentially [1]. It reduces time and work required to produce the restoration while saving its data for later design edits and reproduction [2]. Both ceramic and polymeric materials are used for CAD/CAM fabrication of high-strength full-contour fixed partial dentures (FPD) and frameworks [3]. Despite having superior mechanical strength, ceramics suffer brittleness and higher abrasiveness to opposing natural dentition than polymeric and hybrid-ceramic materials [4]. Internal defects in ceramic restorations can propagate due to intra-oral stresses, leading to restorative failure. This tended to be milder in polymer-infiltrated ceramic network (PICN) materials [5]. CAD/CAM technology uses industrially polymerized prefabricated blocks and discs with a homogeneous structure that increases restorations' mechanical strength and long-term stability [6]. Ceramic, polymeric, and nano-ceramic hybrid composite (NCH) CAD/CAM materials have good stable esthetic qualities; however, polymeric, PICN, and NCH milled restorations are readily repairable intraorally [7]. NCH and PICNs have been reported to possess a flexural modulus that is closer to the natural tooth structure. Compared to conventional methods, processed CAD/CAM materials have fewer internal and external defects created during laboratory steps, such as sintering and polymerization [6]. Niem et al demonstrated that PICN and NCH hybrid materials still suffer from mechanical and esthetic ramifications of water sorption and thermocycling more than ceramic blocks [8]. Lauvahutanon et al also showed d the same results for hybrid and composite CAD/CAM materials but stated that these were within a clinically acceptable range [9]. Thermal challenges intraorally can lead to thermally induced volumetric stresses in dental restorations [8], especially in composite and polymeric materials with varying coefficients of thermal expansion causing detrimental internal stresses [10]. Previous studies indicate increased rate of degradation and decreased strength due to the thermal aging [7, 8, 11]. Egilmez et al demonstrated a significant decrease in flexural strength of CAD/CAM hybrid ceramic materials in response to 3 weeks long water storage at body temperature, autoclaving, and thermocycling for 5000 cycles; corresponding to six months of intraoral use [11]. Additionally, Kim et al demonstrated the deleterious effect of thermal cycling on the flexural modulus and hardness of PICN materials, and the low strength feldspar ceramic blocks [12]. Recently, poly-ether-ether-ketone (PEEK) and fiber-reinforced polymer (FRP) materials have been introduced for framework CAD/CAM construction of FPDs and removable partial dentures (RPDs) [13–16]. PEEK, a thermoplastic polymer, is used as a metal-free bone-like implant material in the medical field since 1998 [15]. Schwitalla et al demonstrated that PEEK material has a flexural strength value exceeding the minimum requirements, making it suitable for dental plastic applications with a strength of at least 65 MPa [15]. However, the material was not compared to other CAD/CAM dental materials. Li et al demonstrated the increased strength of 3D printed PEEK when reinforced with carbon fibers, but the material was not compared to other fiber-reinforced polymers [17].
Table 1. List of manuscript's abbreviations and nomenclature.
Abbreviation | Nomenclature |
---|---|
ANOVA | One-way analysis of variance |
CAD/CAM | Computer-aided design/computer-aided manufacturing |
E | Flexural modulus |
FPD | Fixed partial denture |
FC | Feldspar ceramic |
FRP | Fiberglass-reinforced resin polymer |
FS | Flexural strength |
GLM | General linear model |
GPa | Gega pascals |
h | Hours |
ISO | International Organization for Standardization |
KN | Kilo newtons |
min | minutes |
mm | Millimeters |
MPa | Mega pascals |
NCH | Nano-ceramic hybrid composite |
NTC | Non-thermocycled |
PEEK | Poly-ether-ether-ketone |
PICN | Polymer-infiltrated ceramic network |
PMMA | Poly methy methacrylate |
RPD | Removable partial denture |
s | Seconds |
SiC | Silicon carbide |
TC | Thermocycled |
TEGDMA | Triethyleneglycol dimethacrylate |
Table 1 explains the abbreviations used in the manuscript with their nomenclature, other than those of the tested materials that are explained in table 2.
Table 2. List of CAD/CAM materials used in the study, and their details.
Brand | Abbreviations | Type | Manufacturer | Composition (% weight) | E-Modulus (Gpa) | Flexural strength (Mpa) |
---|---|---|---|---|---|---|
Grandio disc [24] | GR | Nano-ceramic hybrid composite (NCH) | VOCO GmbH, Cuxhaven, Germany | Polymer: 14% UDMA1 + DMA2 Filler: 86% of Nano-hybrid fillers (0.5–3 μm glass ceramic and 0-40 nm SiO2 particles) [20] | 18 | (biaxial) 333 |
Vitablocs Mark II blocks [25] | VB | Feldspar Ceramic (FC) | VITA Zahnfabrik, Baad Sackingen, Germany | Fine-particle feldspar glass ceramic [26] (56%–64% SiO2, 20%–23% Al2O3, 6%–9% Na2O, 6%–8% K2O, 0.3%–0.6% CaO, 0%–0.1% TiO2) | 45 | 154 |
Vita Enamic blocks [27] | VE | Polymer- infiltrated ceramic network (PICN) | VITA zahnfabrik, Baad Sackingen, Germany | Polymer: 14% UDMA1 + TEGDMA3 Filler: 86% ceramic (58%–63% SiO2, 20%–23% Al2O3, 9%–11% Na2O, 4%–6% K2O, 0.5%–2% B2O3, <1% ZrO2, and <1% CaO) | 30 | 150-160 |
JUVORA™ Dental Disc [28] | PK | Polyether ether ketone (PEEK) | JUVORA, Lancashire, UK | 100% PEEK [26] | 4.1[14] | 170 |
Everest C-Temp blocks [29] | CT | Fiberglass- reinforced polymer (FRP) | KaVo Everest, Kaltenbach &Voigt GmbH, Biberach, Germany | Polymer: endless-molecular-chain epoxy resin Filler: Fiberglass fibers | 20 | 500 |
Flexural strength (FS) is paramount to a restoration's long-term survival. In-vitro FS tests can help predict the behavior of the restoration in response to flexion under occlusal stress [18], and durability of CAD/CAM restorations. The appropriate CAD/CAM material for definitive dental restorations is significantly influenced by its resistance to thermal challenges. Palacios et al showed that new hybrid and polymeric CAD/CAM materials assimilate tooth structure's mechanical properties and could be comparable to ceramics when stored in artificial saliva [19]. However, their flexural properties are mostly reported by manufacturers [6]. To ensure that the dental restorative materials chosen are suitable for the task, it is crucial to take necessary measures and carefully consider all relevant options. This involves researching the mechanical properties and characteristics of different materials, their durability, and evaluating the intended use and risks associated with each option. By taking these steps, it is possible to avoid issues or complications that may arise from selecting inappropriate materials for CAD/CAM fabricated FPDs. Limited data exists on the flexural strength and durability of new hybrid and polymeric CAD/CAM materials. Most previous flexural strength studies, compared hybrid ceramic or ceramics to other ceramic materials [8, 20, 21], or compared between different polymeric materials [13, 22, 23], while advising to perform further studies on the flexural properties and behavior of more materials were advised. To the best of the authors knowledge, there have been no prior investigations that have examined the flexural characteristics of four distinct CAD/CAM material categories (including polymeric, fiber-reinforced resin, ceramic, and hybrid materials; those intended for full anatomical restoration and those intended only for framework fabrication.) and evaluated their reaction to thermal aging, while maintaining the same specimen size. The objectives of this study were to investigate the flexural strength and modulus of five commercially available CAD/CAM materials and the effects of thermal cycling on these properties. The null hypotheses were that there were no significant differences in flexural strength and modulus between the different CAD/CAM materials and no significant change in the flexural strength and modulus values due to thermocycling.
2. Method
2.1. Study design
The study was approved and exempt by the research ethics committee of the Faculty of Dentistry in King Abdulaziz University before commencing with the study.
In this in vitro study, flexural strength, and modulus of 5 commercially available CAD/CAM materials for definitive restorations were investigated, two commercially available hybrid ceramic resins, one feldspar ceramic, one polyether-ether-ketone (PEEK) polymeric material for framework construction, and one fiberglass-reinforced (FRP) resin polymer for framework construction of long-term provisional FPDs were investigated. The materials' details, as provided by the manufacturers, are listed in table 2.
100 specimens were prepared. Twenty specimens of each material were prepared. Ten from each group were randomly selected to subdivide the groups into thermocycled (TC) and non-thermocycled (NTC) groups (n = 10). This sample size was calculated using G*Power statistical software [30] (G*Power Ver. 3.0.10, Franz Faul, Universität Kiel, Germany) and the results of a previous study by Sonmez et al [31] to have 80% power (α:0.05) to detect significant differences in flexural strength and modulus. TC specimens were immersed in distilled water and subjected to 10,000 cycles (5 °C–55 °C). All specimens were subjected to a 3-point bending test. Statistical analysis of the results was performed. Figure 1 shows the schematic representation of the study design.
Figure 1. A schematic representation of the study design.
Download figure:
Standard image High-resolution image2.2. Specimen preparation
Bar-shaped specimens (1 × 4 × 13 mm, N = 20) were prepared from each material. The specimen size chosen was in accordance with a previous study [32] and following the modified ISO 6872 standards to determine flexural strength and modulus of tested materials [33, 34], because of ISO 4049 [35] specimen size inappropriateness for the current study due to VB and VE blocks limited size. Specimens were cut using a precision low-speed saw cutting machine (TechCut 4™ precision low-speed saw, Allied High-Tech Products, Inc., Rancho Dominguez, CA, USA) with a 100 mm Super-Thin Rim 0.25 mm thick diamond-coated saw blade (Lapidary saw blade Super-Thin Rim, Jingling, China) under constant water irrigation. According to ISO standards, specimens require finishing and polishing prior to flexural strength testing [34–36]. The specimens were finished and polished using silicon carbide (SiC) paper; 600,800,1200 grits, respectively (sandpaper, Middle East abrasive factory, Riyadh, KSA) under continuous water irrigation using a polishing machine (Struers, Ballerup, Denmark). Past research utilized identical finishing and polishing methods for hybrid, polymer-based, and/or ceramic specimens prior to flexural strength evaluations [22, 37–39]. To ensure accurate reproducible flexural strength values, it was important to confirm uniform specimen size and the absence of dimensional variation [40]. Therefore, the final dimensions were verified with a digital caliper (Vernier caliper; Hi-Wendy, New Taipei, Taiwan). This step ensured that the specimens had the same dimensions and cross-sectional area, preventing any variability that could affect the results. Then the specimens were inspected visually for fractures, defects, and cracks. The defective specimens were discarded. Figure 2 shows one of the finished specimens.
Figure 2. An example of a finished bar-shaped material specimen.
Download figure:
Standard image High-resolution image2.3. Thermal cycling
Ten specimens were randomly selected from each material group and subjected to a thermocycling process of 10,000 alternate thermal cycles between 5 and 55 °C in a distilled water bath in an automatic thermocycling machine (Thermocycler 1100/1200, SD Mechatronik, Feldkirchen- Westerham, Germany). The thermocycler used in this study is shown in figure 3. Each cycle lasted for 40 s:15 s in a 5 °C bath, 5 s to transfer the specimens between the two baths , 15 s in a 55 °C bath, and 5 s to transfer the specimens back to the 5 °C bath. The water tanks were refilled daily with approximately 1 liter of distilled water to compensate for overnight water evaporation. The thermocycling machine was checked regularly to ensure the regular movement of specimens between water tanks, sufficient water, and stable temperatures in each water bath.
Figure 3. Thermocycler 1100/1200, SD Mechatronik, Feldkirchen- Westerham, Germany.
Download figure:
Standard image High-resolution image2.4. Flexural strength (FS) and flexural modulus (E) determination
All specimens were subjected to a 3-point bending test using an Instron universal testing machine (5940 Series, Instron, Norwood, Massachusetts, USA). The span distance was 12 mm with a crosshead speed 0.5 mm min−1 in 2 KN cell. NTC Specimens were tested after 24 h of dry storage following their preparation. TC specimens were tested after 24 h of dry storage following thermocycling. All the specimens were subjected to static loading in the machine until complete fracture. PK specimens were loaded until complete specimen deformation. Figure 4 shows the Instron universal testing machine used in the study.
Figure 4. Instron universal testing machine with a sample undergoing 3-point bending test.
Download figure:
Standard image High-resolution imageValues recorded by 3-point bending test were used to calculate FS, in MPa, and E , in GPa, according to the following formulas (ISO 6872-2015, [34]):
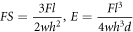
Where:
= maximum applied load (N),
= distance between the supports (12 mm),
= width of the specimen(4 mm),
= height of the specimen (1 mm),
= deflection at maximum load
(mm).
2.5. Statistical analysis
The results were analyzed using SPSS statistical software (SPSS, Version 22, IBM Corporation, New York, USA). In each group, the following descriptive statistical parameters of the flexural strength (FS) and modulus (E) were calculated: mean, standard deviation, minimum, and maximum. The mean values of FS and E were analyzed using a one-way analysis of variance (ANOVA) to detect significant differences between the tested material groups. A paired sample t-test was used to compare each material group's mean flexural strength and modulus before and after thermocycling. A general linear model (GLM) repeated measure ANOVA, and Multiple pairwise comparisons (Bonferroni) were conducted to compare the mean flexural strengths and moduli of the TC and NTC material groups at a 5-percent significance level (p = 0.05).
3. Results
Shapiro-Wilk normality test showed a normal distribution of the values for all materials before and after thermocycling (p > 0.05). Thus, parametric tests were applied (p = 0.05). Descriptive statistics for the flexural properties of all groups before and after thermocycling are detailed in table 3.
Table 3. Descriptive statistics of flexural properties of all material groups.
FS (MPa) | E (GPa) | |||
---|---|---|---|---|
Material group | Thermocycling Status | n | Mean (SD) | Mean (SD) |
GR | NTC | 10 | 385.14 (90.73) | 26.37 (3.75) |
TC | 10 | 369.52 (84.93) | 23.37 (3.73) | |
VB | NTC | 10 | 189.92 (32.53) | 62.93 (10.64) |
TC | 10 | 194.07 (52.51) | 44.50 (22.57) | |
VE | NTC | 10 | 224.66 (25.51) | 46.90 (6.21) |
TC | 10 | 184.00 (18.10) | 34.77 (6.81) | |
CT | NTC | 10 | 924.88 (120.11) | 33.46 (7.02) |
TC | 10 | 848.40 (151.37) | 26.53 (6.33) | |
PK | NTC | 10 | 309.56 (46.84) | 5.76 (1.35) |
TC | 10 | 277.24 (75.28) | 5.14 (1.10) |
3.1. Flexural strength results
One-way ANOVA revealed a statistically significant difference in the mean NTC FS values between the material groups (p < 0.001). CT had the highest FS (NTC = 924.88 ± 120.11 MPa, TC = 848.40 ± 151.37 MPa) followed by GR (NTC = 385.13 ± 90.73 MPa, TC = 354.56 ± 43.10 MPa) and PK (NTC = 309.56 ± 46.84 MPa, TC = 277.24 ± 75.28 MPa). GLM ANOVA showed a significant effect of time (TC) as a main effect on the material (p = 0.05); therefore, at least one material group was significantly affected by time. The interaction between time and material was not significant (p = 0.59). The type of material as the main effect was significant (p < 0.001). Paired samples t-test showed a statistically significant reduction in the mean FS of VE after TC (p = 0.002). Figure 5 shows the mean FS values of the materials before and after thermocycling with significant t-test results.
Figure 5. Flexural strength (FS) means of all material groups before (NTC), and after (TC) thermocycling. * Paired samples t-test significant difference (P = 0.002) between NTC and NTC groups.
Download figure:
Standard image High-resolution imageBonferroni pairwise comparison between every pair of NTC and TC material groups is presented in table 4. VB had a statistically significantly lower FS than GR, CT, and PK (p < 0.001, p < 0.001, and p = 0.006) (NTC = 189.92 ± 32.53 MPa, TC = 194.07 ± 52.51 MPa). GR material group (NTC = 385.13 ± 90.73 MPa, TC = 354.56 ± 43.10 MPa) exhibited a significantly higher FS than VB (NTC = 189.92 ± 32.53 MPa, TC = 194.07 ± 52.51 MPa) and VE (NTC = 224.65 ± 25.51 MPa, TC = 184.00 ± 18.11 MPa). The CT specimens (NTC = 924.88 ± 120.11 MPa, TC = 848.40 ± 151.37 MPa) had a significantly higher fracture strength than the rest of the material groups (p < 0.001). There was no statistically significant difference between the material groups regarding mean FS change due to thermocycling (TC–NTC) (p = 0.59).
Table 4. Bonferroni comparisons of the material groups' flexural strength (FS).
95% Confidence interval | ||||||
---|---|---|---|---|---|---|
Material group | Material group | Std. error | Mean difference | P value | Lower bound | Upper bound |
GR | VB | 195.23 | 32.60 | <0.001* | 99.00 | 291.45 |
GR | VE | 160.49 | 32.60 | <0.001* | 64.26 | 256.71 |
GR | CT | −539.74 | 32.60 | <0.001* | −635.96 | −443.51 |
GR | PK | 75.58 | 32.60 | 0.250 | −20.64 | 171.81 |
VB | VE | −34.74 | 32.60 | 1.000 | −130.96 | 61.49 |
VB | CT | −734.96 | 32.60 | <0.001* | −831.18 | −638.74 |
VB | PK | −119.64 | 32.60 | 0.006 | −215.87 | −23.42 |
VE | CT | −700.22 | 32.60 | <0.001* | −796.45 | −604.00 |
VE | PK | −84.90 | 32.60 | 0.124 | −181.13 | 11.32 |
CT | PK | 615.32 | 32.60 | <0.001* | 519.09 | 711.54 |
3.2. Flexural modulus results
One-way ANOVA revealed a statistically significant difference in the flexural modulus (E) between the groups (p < 0.001). VB had the highest E (NTC = 62.93 ± 10.64 GPa, TC = 44.5 ± 22.57 GPa) followed by VE (NTC = 46.9 ± 62.1 GPa, TC = 34.77 ± 68.14 GPa), then CT (NTC = 33.46 ± 70.15 GPa, TC = 26.53 ± 6.33 GPa). GLM ANOVA showed a significant effect of time (TC) as a main effect on the material (p < 0.001); therefore, at least one material group was significantly affected by TC. The interaction between time and material was statistically significant (p = 0.03). The type of material as main effect was statistically significant (p < 0.001). Paired samples t-test showed that VE and CT had a statistically significant reduction in E after TC (p < 0.01 and p = 0.013, respectively). Figure 6 shows the mean E values of the different materials before and after TC with significant t-test results.
Figure 6. Flexural modulus (E) means of all material groups before (NTC), and after (TC) thermocycling. * Paired samples t-test significant difference between NTC and NTC groups.
Download figure:
Standard image High-resolution imageBonferroni multiple pairwise comparisons between the mean E values of the material groups are detailed in table 5. VB had a significantly higher E than GR and PK (P < 0.001). GR (NTC = 26.37 ± 3.75 GPa, TC = 23.37 ± 3.7 GPa) had a significantly lower mean E than VB but a significantly higher than PK (p = 0.008). PK (NTC = 5.77 ± 1.35 GPa, TC = 5.14 ± 1.1 GPa) had a statistically significantly lower mean E than VE and CT. CT's mean E was significantly higher than GR (26.37 ± 3.75 GPa) and significantly lower than VB (62.93 ± 10.64 GPa). One-way ANOVA showed that at least one of the mean values of E change (TC-NTC) for a material group was significantly different from that of the other groups (p = 0.03). Bonferroni multiple comparisons revealed a significantly greater change in ΔE (TC-NTC) in VB than in PK (p = 0.046).
Table 5. Bonferroni multiple pairwise comparisons of the flexural modulus between groups.
95% Confidence interval | ||||||
---|---|---|---|---|---|---|
Material group | Material group | Mean difference (MPa) | Std. error | P Value | Lower bound | Upper bound |
GR | VB | −36.56 | 2.95 | <0.001* | −45.26 | −27.86 |
GR | VE | −20.53 | 2.95 | <0.001* | −29.22 | −11.83 |
GR | CT | −7.09 | 2.95 | 0.202 | −15.7 | 1.60 |
GR | PK | 20.61 | 2.95 | <0.001* | 11.91 | 29.30 |
VB | VE | 16.03 | 2.95 | <0.001* | 7.34 | 24.73 |
VB | CT | 29.46 | 2.95 | <0.001* | 20.77 | 38.16 |
VB | PK | 57.17 | 2.95 | <0.001*,s | 48.47 | 65.86 |
VE | CT | 13.43 | 2.95 | <0.001* | 4.73 | 22.13 |
VE | PK | 41.13 | 2.95 | <0.001* | 32.44 | 49.83 |
CT | PK | 27.70 | 2.95 | <0.001* | 19.01 | 36.40 |
*statistically significant difference in flexural modulus (E) between materials, s statistically significant difference in flexural modulus change (ΔE) between materials.
4. Discussion
Computer-aided design/computer-aided manufacturing (CAD/CAM) systems have evolved into efficient modeling and manufacturing methods combined with developing composite hybrid materials with better mechanical properties and easier adjustability and repairability [5]. Posterior teeth and restorations are subjected to multidirectional intraoral forces ranging from 150–665 N up to 1,221 N in bruxism cases [41]. For a long positive prognosis, materials for CAD/CAM definitive restorations must tolerate these forces and other intraoral factors. The flexural strengths and moduli of five tooth-colored CAD/CAM materials were tested: NCH (GR), PICN (VE), PEEK (PK), FRP (CT), and Feldspar Ceramic (VB). Both hypotheses, that there were no significant differences in flexural strength and modulus between the different CAD/CAM materials, and that there was no significant change in the flexural strength and modulus values of the tested materials after thermal cycling, were rejected owing to the presence of significant differences in flexural strengths and moduli between the materials and in response to thermaocycling (time) using ANOVA (p < 0.001).
In vitro tests of newly introduced materials under controlled simulated conditions provide insight into materials' clinical suitability and durability [10]. According to ISO 4049, occlusal-surface-restoring resin materials must have a flexural strength of at least 80 MPa [36]. The flexural strength values obtained in this study were higher than those provided by the manufacturers. This variation could correspond to the difference in the bar specimen sizes that would affect the span between the supports in the 3-point-bending test [4]. Owing to the limited size of the CAD/CAM blanks, the test specimens were cut to smaller dimensions than ISO4049 standards but followed those used in previous studies [9, 32]. In this study, CAD/CAM blanks were cut under copious water cooling to avoid heat generation within the tested material. Industrially polymerized CAD/CAM blanks have more uniform, less porous, and stronger structures than their conventional counterparts [42].
CT exhibited significantly higher flexural strength than other PICN (VE), NCH composite (GR), PK, and feldspar ceramic (VB) materials (P < 0.001). Few clinical studies have reported using CT CAD/CAM materials marketed by manufacturers for long-term provisional FDPs frameworks. FRP dental materials have three components: matrix, dispersed phase (usually glass fibers), and interphase. The matrix bears external stress and transfers it to the dispersed phase, which in turn reinforces the strength of the material [43]. The tested CT polymer material contains long continuous molecular chains and a significant amount of dense high-strength glass fibers that are industrially incorporated into the block structure, which enhances its strength to over 500 MPa according to the manufacturer's data. This particular material boasts a very low level of chemical solubility, measuring in at a mere 0.55 μg cm−3, and a water absorption rate of only 9.6 μg cm−3 [29]. These unique properties work together to maintain its durably high strength during extended intraoral use periods. The strength of dental polymeric materials has been reported to increase with the incorporation of glass fibers, as shown in previous studies [44–46]. Previous studies by Suzaki et al demonstrated the superior flexural strength of CAD/CAM FRP, particularly if the fiber directions were identified [44, 47], which is supported by the results of the current study. This is in accordance with the results of a recent study by Babaier et al that concluded the higher flexural strength of two similar FRP CAD/CAM materials (ranging between almost 400-500 MPa) compared to PEEK (around 200 MPa) [48]._NCH blocks (GR) are relatively new and marketed for their superior mechanical and esthetic properties, requiring no firing or lamination. The flexural strength of GR was significantly higher than PICN (VE) and feldspar ceramic (VB) (p < 0.001). Sakai et al showed, using artificial intelligence, a positive correlation between UDMA content and flexural strength, which could explain the higher flexural strength of GR than VE [18]. Lucsanszky et al showed that dispersed-filler resin composite blocks, similar to GR, had higher flexural strength than VE [4]. This could be due to the high inorganic filler content of GR (86% w/w high inorganic fillers in the polymer matrix), the different filler geometries, or the filler-matrix network arrangement. Alamoush et al showed a correlation between filler content of NCH and PICN and positive mechanical properties [26]. VB had the lowest flexural strength (p < 0.001). This correlates with the high brittleness index of the material observed in a previous study [49]. Hensel et al showed high fracture loads of the material when used to mill 3-unit FDPs; indicating the suitability of GR for the fabrication of restorations in high-stress areas [50]. In this study, GR and PK had no significant difference in FS between them (p = 0.25) but were significantly higher than VB and VE (p < 0.001). PEEK was introduced for the fabrication of metal-free removable [51, 52] and fixed [53] partial denture frameworks because of its flexibility, biocompatibility, and tensile properties, which are close to those of bone and dental hard structures [14, 26]. However, the veneering material type Field significantly affects the final strength of PEEK FPDs [54]. GR can be milled to full contour and thus is not negatively affected by the mechanical properties of a weaker veneering composite. All the tested materials had flexural strength values above ISO 4049 [36]-required 80 MPa before and after aging. Thus, the tested hybrid and polymeric materials are suitable for milling FPD with occlusal components.
This study's flexural moduli (E) significantly differed among the materials. VB material exhibited the highest E (p < 0.001), which was closer to that of enamel than dentin [9]. The ceramic materials' stiff and brittle nature, negatively affects their durability when subjected to repetitive flexural forces [49]. The flexural modulus of the VE PICN was significantly higher than GR (p < 0.001). Alamoush et al showed that ceramic materials have the highest elastic modulus and that PICN has a higher elastic modulus than other polymeric and ceramic hybrid materials [26]. The different polymer manufacturing and ceramic networking techniques could explain the higher modulus and stiffness of VE with GR [26]. GR and CT (26.4 and 33.5 GPa, respectively) had an E that was not significantly different from each other but was significantly lower than VB and VE. Their moduli were closer to dentin (approximately 30 GPa [55]) than VE and VB, which could make their bending behavior closer to the surrounding tooth structure, thus, a longer intraoral service. Suzaki et al showed no significant difference in the flexural modulus of a CAD/CAM FRP-like CT compared to other fiber-integrated composites, and that it was not affected by the direction of the fibers within the material [44]. Fiber integration was found mechanically beneficial by Bechir et al [43]. PK had the lowest E compared with the other materials (p < 0.001). This corresponds to the high bending potential of the material with stiffness values similar to that of polymethylmethacrylate (PMMA), making the material suitable as a framework for long-term FPDs [56].
Thermocycling has been used to simulate the hydrolytic and thermal degradation aging processes that occur intraorally over time. This study subjected the materials to 10,000 cycles between 5 and 55 °C in distilled water, corresponding to almost one year of intraoral use [57]. In this study, there was a significant effect of thermocycling (time) as the main effect, and the interaction between time and material was also significant (p < 0.001 and p = 0.03, respectively); however, the effects were not the same for all tested materials. Thermocycling caused a significant reduction in FS and E of VE (p = 0.002) and E of CT (p = 0.01). VE is a ceramic-polymer hybrid material with a ceramic network accounting for 86% of its weight and a reinforced polymer network making up the remaining 14% [27]. This combination provides a balance of high load-bearing capacity, efficient absorption of masticatory forces, and elasticity [58]. Thermal-induced separation of the fillers from the resin matrix can cause this reduction. Additionally, the different thermal coefficients of expansion of the fillers and matrix could cause the propagation of microcracks within the resin-based composites, reducing their strength [59]. Lucsanszky et al showed thermocycling's negative effect on FS of hybrid (i.e. GR) and PICN (i.e. VE) CAD/CAM materials, but not on the flexural modulus of VE [4]. The current study showed a decrease in VE modulus, similar to a study using 5,000 thermocycles [60]. Egilmez et al showed that the FS of VE deteriorated with thermocycling [11]. In this study, FS and E of GR were not significantly affected by thermocycling (p = 0.67,p = 0.17, respectively), similar to the machinable composite material tested by previous studies [50, 61] ; but the flexural modulus of VE decreased significantly (p = 0.003). The thermocycling-induced significant reduction in VE's (from about 46.9 to 34.77 GPa) and CT's ( from about 33.46 to 26.53 GPa) flexural moduli indicated that VE becomes softer and less stable intraorally with time, and stiff strong CT softens with time becoming less stiff and rigid. The resin matrix of these materials may absorb water during thermocycling, thereby causing the VE and CT network to expand and reduce the frictional forces of polymer chains [31, 62]. This, in turn, can weaken the interfacial silane coupling agent that links the resin matrix and inorganic fillers, ultimately leading to the material's softening [31]. VE is particularly susceptible to degradation due to its organic matrix containing the diluting monomer, triethyleneglycol dimethacrylate (TEGDMA). TEGDMA has a molecular weight of 286 g mol−1, a low viscosity of 0.0014 Pa s, and a higher potential for water sorption and subsequent degradation than other monomers [63, 64]. This results in softening of the material after thermocycling. [63, 65]. Sonmez et al demonstrated the deterioration of the homogonous structure of VE due to thermal cycling resulting in the material reduction in flexural modulus [31], which supports the results of the current study. The lack of a significant effect of thermocycling on GR and PK's FS and E could be due to their ability to dissipate energy elastically and plastically. In this study, VB was not affected by thermocycling, which is in accordance with previous studies [8, 61]. This can be due to the ceramics' nature, where thermal aging-induced crystal/matrix thermal expansion mismatches strengthen the material rather than impair it [66]. In this study, PK demonstrated no significant reduction in flexural strength values after thermocycling (p = 0.33), which is in accordance with the results of Niem et al [8]. Polymer networks have the potential to absorb water, leading to a deterioration of the material's mechanical properties and changes in their microstructure [67]. However, this potential did not appear to affect the flexural properties of PK, which is composed entirely of PEEK polymer, in response to thermocycling. Liebermann et al demonstrated the lower water sorption and solubility of PEEK polymers compared to other CAD/CAM polymer and hybrid materials including VE [56], which would explain the nonsignificant effect of thermocycling on the flexural strength of PK in the current study. Long-term studies with more factors considered are recommended.
5. Conclusions
The present study delves into the flexural mechanical properties of four categories of dental restorative materials developed for dental computer-aided design and computer-aided manufacturing (CAD/CAM) technology. CAD/CAM fabricated restorations are subjected to a range of stresses and temperatures in the oral environment, which can significantly compromise their mechanical properties over time. It is essential to understand the extent of these changes and their potential implications for the longevity and performance of dental restorations. Interestingly, ceramic, hybrid, and polymeric materials are not all created equal when it comes to flexural strength. In fact, it was noted in the current study that HCN GR, PEEK, and FRP materials tend to have higher flexural strength compared to other types of CAD/CAM feldspar and hybrid ceramic materials currently available. This finding could have important implications for various dental applications that rely on strong and durable restorative treatments. While the CAD/CAM technology and materials discussed in the study have shown promising results, it is important to note that there may be limitations and potential drawbacks to their use for dental restorations. One potential limitation is the cost of the technology, which may not be feasible for all dental practices and/or patients. Additionally, there may be a learning curve for dental practitioners and technicians to become proficient in using these materials and technology. As with any new technology or material, there may also be unforeseen complications or challenges that arise with its use. It is important for dental professionals to carefully consider these factors before choosing the restorative fabrication and materials into their practice. Additionally, the current study relied on in-vitro testing using the 3-point bending test. It was suggested in the literature that diametral flexural strength testing might be more accurate. Further studies using different testing methodologies and longer thermocycling duration are recommended. In vivo tests are time-consuming, involving the interplay of many intraoral factors [6]. In vitro simulation of these factors, such as thermocycling, sheds light on their effects on the material's mechanical properties [8, 10, 11]. Therefore, further in-vivo testing would be advisable to shed light on the actual durability of the restorations. It is hoped the current study will help clinicians make an informed clinical decision in choosing the material by knowing the difference in the flexural strength between the different CAD/CAM composites and ceramics materials, as well as to stimulate further research on the new CAD/CAM nano composite hybrid material. Within the limitations of the current study, it can be concluded that Grandio disc for CAD/CAM fabrication, which is a nano-ceramic hybrid composite material, exhibits a higher stable flexural strength than polymer-infiltrated ceramic network materials and feldspar ceramics, and is comparable to that of PEEK polymeric materials for framework construction. Therefore, they may be suitable for cases with higher stress loads. Additionally, the thermal cycling effect on the flexural strength and modulus of the CAD/CAM materials differed between the materials.
Acknowledgments
The authors would like to acknowledge with thanks to The Advanced Technology Dental Research Laboratory (ATDRL), Faculty of Dentistry, King Abdulaziz University, for their technical support.
Data availability statement
All data that support the findings of this study are included within the article (and any supplementary files).
Institutional review board statement
Ethical approval was obtained from the research ethics committee of the Faculty of Dentistry at King Abdulaziz University, IRB protocol 105-10-22.
Conflicts of interest
The authors declare no conflict of interest. The funders had no role in the design of the study; in the collection, analyses, or interpretation of data; in the writing of the manuscript; or in the decision to publish the results.