Abstract
In this manuscript one-dimensional (1D) photonic biosensor (PQ)NGDG(PQ)Ncomposed of phase change material (PCM) germanium antimony telluride (GST) has been studied in visible region of electromagnetic spectrum. This design is capable of label free recognition of reproductive hormones of female which are significant during reproductive process such as menstruation and parturition. The proposed structure is composed of an air cavity separated by two buffer layers of GST material. The GST buffer layers have been used to improve the sensing performance of the structure. The modified cavity associated with buffer GST layers is sandwiched between two 1D photonic structures (PS) (PQ)N. Both 1D PS are consisted of alternate material layers of SiO2 and Si of period 5. The transmission spectra of proposed design have been obtained by using transfer matrix method and MATLAB software. In this work the performance of the devise has been investigated for normal and oblique incidence corresponding to TE wave only under the influence of change of phases of GST. The mainstay of this research is focused on the tunable performance of proposed bio-sensing design due to switching between amorphous phase (aGST) and crystalline phase (cGST) of GST. Moreover how the change in the thickness of cavity region as well as angle of incidence corresponding to TE wave affects the performance of the design has also been studied. The sensitivity, quality factor and figure of merit values of the design have also been studied to get deep insight about the sensing capabilities of the proposed design under the influence of crystalline and amorphous phases of GST. Thus due to simple architecture and excellent switchable and reconfigurable characteristics, our structure works efficiently in industrial and biomedical refractive index based sensing applications.
Export citation and abstract BibTeX RIS
1. Introduction
In recent decades photonic structures have attracted worldwide attention of researchers due to their unique electromagnetic properties and applications in the field of medical diagnostic, engineering optics, food safety testing, biochemical analysis and environmental monitoring [1, 2]. These structures provide a powerful platform for designing of photonic devices which could find diverse range of optical and technological applications owing to tremendous ability of manipulating amplitude, polarization and phase of electromagnetic waves [3–5]. The landmark achievements of two renowned scientists Yablonovitch and John who discovered photonic crystals (PCs) in 1987 [6, 7] open a door for developing engineered photonic devices like topological waveguides [8], photonic crystal fibers [9], high efficiency LEDs [10], absorbers [11], optical couples [12] and photonic biosensors of high sensitivity and resolution [13–15]. Unfortunately most of these devices are made up of passive components and have fixed optical response which gradually impeded their technological use especially in the optoelectronic and integrated photonics where active optical properties are essential requirements [16]. For fulfilling these requirements nowadays people have started the use of active materials like electrically tunable dielectrics [17], strain sensitive materials [18], micro-electro-mechanical systems (MEMS) based technology [19] in the fabrication of photonic devices to induce active feature like tunability i.e. altering a spectrum of photonic structures by means an electrical, optical, thermal or mechanical tuning mechanism externally. In 1998 Figotin et al in their early work showed how ferroelectric and ferromagnetic materials can be used to construct tunable PCs because of electric and magnetic fields dependent permittivity and permeability functions of ferroelectric and ferromagnetic materials respectively [20]. Currently available nanophotonics-inspired approaches of constructing tunable PCs are mainly based on metallo-organic frame work in which tunability is achieved by isomerization [21], soft material based polymer structures in which spectrum is tuned by hydrostatic forces [22], liquid crystals [23] based tunable PCs and tunable acousto-optical photonic structures [24] etc All such technologies are not deemed fit for designing tunable PCs which have suitable applications in the field of integrated photonics as well as optoelectronics due to their complexity and compatibility issues with the presently available micro and nano fabrication techniques [16].
Recently phase change materials (PCM) [25] have emerged as one of an efficient and earth abundant materials to be used as an alternative of active material for fabricating tunable photonic devices due to their compatibility and simplicity with thin film deposition techniques, better thermal stability, fast response time and robust switching speed [26]. The chalcogenide alloys exemplified by the GeSbTe (GST) family are one of PCMs which is composed of germanium (Ge), selenium (Se) and tellurium (Te) three elements of group 16 of periodic table and have large refractive index contrast between amorphous and crystalline phases [27]. The chalcogenides can be doped easily with rear-earth element which make them most suitable candidate for designing of wide range of photonic devices like reconfigurable photonic memories, nonvolatile display, all-optical computers and photonic switches [28]. The appropriate application of electrical [29], thermal [30] or optical [31] stimulus can allow the GST alloys to toggle between amorphous and crystalline phases. This special feature of GST alloys can be used for developing variety of active photonic devices equipped with switchable and reconfigurable optical properties such as Fresnel zone plate [31], tunable meta-surfaces for controlling wavefronts [1, 22], thermal emitters [30] and absorbers [32]. From last few years several researchers have shown their keen interest for realization of GST based switchable spin–orbit interaction meta-surfaces, tunable nanophotonic devices with the help of meta-surfaces, photonic sensors and modulators [16–26] etc Thus GST is most suitable choice for designing of tunable photonic devices of high degree of freedom which may be used for label-free sensing application in contrast to mechanically tuned liquid crystals and other optical devices. For example Gerislioglu et al reviewed the resent achievements of advanced and multifunctional nanophotonic devices due to the application of PCMs and their compounds [33]. Moreover how to extract distribution of temperature within memory cell composed of PCMs during crystallization has been studied by Bakon et al [34].
Motivated by aforementioned piece of excellent research work [1–16] and importance of GST material [26–32] in this manuscript 1D photonic biosensor capable of detecting minute refractive index changes in blood samples containing progesterone and estradiol reproductive hormones of different concentration levels in presence of cGST and aGST phases of Ge2Sb2Te5 material, has been studied. This design successfully measures the shift in location of the defect mode within PBG of proposed structure depending upon respective change in refractive index of blood samples containing reproductive hormones under cGST and aGST phases both, of Ge2Sb2Te5 material. The comparison between the positions of defect modes depending upon the variation in refractive index of blood samples of different concentration levels under influence of crystalline and amorphous phases will give us the information about the presence of reproductive hormones. Recently Ayyanar et al suggested a reconfigurable 1D photonic biosensor consisting of GST material which can be used for detection of progesterone and estradiol reproductive hormones [35]. But the defect mode of that design shifts from visible to infrared region of investigation when GST material is switched from amorphous to crystalline phase respectively [35]. We have strong belief that phase dependent tuning of defect mode inside PBG from visible to infrared region limits the performance of their design. The purpose of this work is to address this issue and suggest alternative design in which movement of the defect mode is solely restricted in visible region during transition of GST material between amorphous and crystalline phases. To the best of our knowledge 1D photonic bio-sensing structure (PQ)5(GDG)(PQ)5 consisting of defect with GST buffer layers has been rarely used for detecting progesterone and estradiol reproductive hormones of different concentration levels in visible region. The architecture of the proposed work is as follows: section 2 deals with structural blueprint of the proposed photonic design. The theoretical modeling of the problem has been discussed in section 3. In section 4 we have presented results and discussions pertaining to the work.
2. Theoretical formulation of proposed design
The proposed one-dimensional photonic bio-sensing device (PQ)N GDG(PQ)N is formed by combining two identical 1D photonic structures (PQ)N through the modified defect layer D whose both sides are coated with two identical buffer layers G made up of phase change chalcogenide material Ge2Sb2Te5 [35] as shown in figure 1.
Figure 1. The schematic diagram of 1D photonic biosensor (PQ)5GDG (PQ)5. Here letters P, Q, G and D are being used to represent SiO2, Si, GSTand cavity layers of the structure respectively.
Download figure:
Standard image High-resolution imageThe cavity layer D is infiltrated by separate blood samples containing progesterone and estradiol reproductive hormones of different concentrations under investigation. The plane electromagnetic waves are allowed to fall on the structure at angle of incidence θ0 with respect normal which is z-axis in our case. Both 1D PSs are composed of alternate material layers P and Q of period number N. The refractive indices and thicknesses of layers P, Q and D are nA, nB, nD, dA, dB and dD respectively. In our architecture, layer G is made up of phase change material which has distinct refractive indices na and nc depending upon the amorphous and crystalline phases of GST material respectively. The theoretical results have been obtained with the help of transfer matrix formulation [35–38]. The computations have been performed by using MATLAB software. The total transfer matrix of entire structure representing the interaction between the incident electromagnetic radiation and the structure is defined as

where M11, M12, M21 and M22 are representing the elements of total transfer matrix of whole structure. The characteristic matrix of layers P, Q, G and D of materials SiO2, Si, GST and air respectively are described below. Here it should be kept in mind that the switching between amorphous to crystalline and crystalline to amorphous phases will change the entire characteristic matrix of GST material due to change in effective refractive index of GST material in amorphous to crystalline phases.
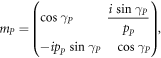
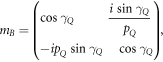
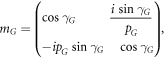
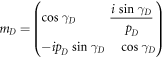
The phase difference γ associated with layers P, Q, G and D are defined as

Here
and
are ray angles inside layers P, Q, G and D respectively. The values of p associated with layers P, Q, G and D are given as
and
respectively corresponding to TE wave.
The transmission coefficient of the proposed bio-sensing design (PQ)N GDG(PQ)N based on Chebyshev polynomial of second kind can be evaluated as

Here the values of p corresponding to TE wave inside incident and exit media are defined as and
respectively. Finally transmittance of the proposed 1D photonic biosensor design (PQ)N
GDG(PQ)N
can be obtained as

3. Result and discussions
3.1. Structural design
We are proposing a 1D photonic bio-sensing structure (PQ)N GDG(PQ)N capable of detecting reproductive hormones of female as depicted in figure 1. The proposed photonic structure (PS) is composed of two identical 1D PSs (PQ)N and the modified defect layer D whose both sides are coated with two identical buffer layers G made up of phase change chalcogenide material Ge2Sb2Te5. This modified three layer assembly GDG is embedded between 1D PSs (PQ)2N to configure proposed 1D photonic bio-sensing structure (PQ)N GDG(PQ)N . The proposed architecture integrated with GST material induces switchable spectral response and also prevent direct exposure of Si material layer with air and water which in turn restricts the possibility of oxidation of silicon in presence of blood sample to be investigated. The detection of reproductive hormones in the blood samples of female patient has been done by pouring it inside cavity region D of air which is sandwiched between buffer layers of GST material. The letters P and Q are used to represent SiO2 and Si material layers respectively of 1D PS (PQ)N . The letter N symbolizes the period number of the structure which is fixed to 5. The entire 1D photonic bio-sensing structure has been kept in air. The thickness of layers P, Q, G and D of the proposed design are optimized to dP = 380 nm, dQ = 260 nm, dG = 20 nm and dD = 320 nm respectively which yields wider PBG and high sensitivity value of the proposed design. The investigations of proposed research work has been carried out in the region of investigation 600 nm to 770 nm in which the refractive indices of materials SiO2 and Si are considered as nP = 1.45 and nQ = 3.3 respectively.
3.2. Determination of refractive index of phase change material based on chalcogenide alloy of GeSbTe (GST) material
Phase change material exhibits unconventional remarkably different optical properties between amorphous and crystalline state under the influence of appropriate thermal, optical or electrical stimuli. In this work we have analyzed the performance of proposed design by considering the experimentally obtained complex refractive index values of Ge2Sb2Te5 material under amorphous and crystalline phases both with the help of spectroscopic ellipsometry technique. In this experimental technique thin film of GST material of thickness 20 nm is grown over silicon wafer and by applying Tauc-Lorentz model the complex refractive index values of GST material in terms of wavelength dependent real and imaginary parts are obtained for amorphous and crystalline phases both. Conceptual visualization of this statement has been shown in figure 2 which depicts real and imaginary parts of complex refractive index of GST material in amorphous and crystalline phases both. Here notations naGST, ncGST and KaGST, KcGST are being used to represent real and imaginary parts of complex refractive index of GST material in amorphous and crystalline phases respectively.
Figure 2. Real and imaginary parts of refractive index of GST material under amorphous and crystalline phases both. These values have been obtained by applying polynomial curve fitting technique on experimental data.
Download figure:
Standard image High-resolution imageThere are several methods by which phase transition between amorphous to crystalline and back in GST materials can be achieved. For example thermal heating through laser pulse of duration micro or nano seconds can be used for robust switching between amorphous to crystalline phases of GST material. Other conventional methods like optical or electrical stimuli may also be used for switching between amorphous and crystalline phases of GST material. The large value of thermal stability and high switching speed makes the GST material as a promising candidate for designing of rewritable and nonvolatile optical devices which in turn may be useful to realize reconfigurable and switchable photonic devices. In this study the phenomenon of phase change in GST material can be easily achieved by applying laser pulse of short during only over buffer layers made up of phase change material. Moreover the optical properties of materials SiO2 and Si used in this design along with blood samples containing reproductive hormones will not be affected by the application of laser pulse of short duration on Ge2Sb2Te5 material for switching between aGST and cGST phases.
The experimentally obtained complex refractive index values of Ge2Sb2Te5 material under amorphous and crystalline phases [35] have been used to develop theoretical relations giving real and imaginary values of Ge2Sb2Te5 material under amorphous and crystalline phases with the help of polynomial curve fitting of degree 3 and 4 to ensure higher accuracy between experimental and theoretical results. The following expressions 4 and 5 are giving theoretical values of complex refractive index of Ge2Sb2Te5 material under amorphous and crystalline phases used in this study respectively.


Here naGST and KaGST are representing real and imaginary parts of complex refractive index of GST material in amorphous phase whose curve fitting expressions are as under


Similarly ncGST and KcGST are representing real and imaginary parts of complex refractive index of GST material in crystalline phase as under


Figure 2 shows the variation of naGST, KaGST, ncGST and KcGST with respect to wavelength. There is a pronounced contrast of complex refractive index properties of GST material between amorphous and crystalline phases in near infrared region. This property of change in refractive index of GST material under amorphous and crystalline phases opens new gateway for designing of reconfigurable and switchable photonic biosensors with ultra high sensitivity based on GST materials.
3.3. Determination of refractive index of progesterone and estradiol reproductive hormones along with their significance
In this section we describe the refractive index formulae to determine refractive index values of progesterone and estradiol reproductive hormones in females. These values are dependent on their respective concentration level in blood samples. Progesterone is a type of steroid hormone which needs to be investigated during menstrual cycle disorder as well as early stages of pregnancy. The role of progesterone in females is more significant during reproductive process such as menstruation and parturition. This hormone has the ability to release egg from ovary of women when it is fertilize by sperm. Besides this the production of milk in mammary glands located inside breasts of females begins when progesterone hormone combines with other hormones. The progesterone is also responsible for developing fetus in uterus during late pregnancy. Moreover estradiol is a type of estrogen which is considered to be female sex hormone. Its main purpose is to establish and then retain the reproductive system. The increase in estradiol level causes the ripeness and release of the eggs during the menstrual cycle. These hormones are also responsible for the thickening of the uterus lining during implantation of fertilized egg. This hormone is very essential in males' sexual function which includes libido, erectile function, and spermatogenesis.
The refractive index of progesterone and estradiol hormones depending upon their concentration levels in blood sample has been theoretically obtained with the help of expressions 10 and 11 respectively as given below.


Here c is the concentration level of progesterone and estradiol hormones in blood sample in unit of nmol l−1. We have used experimentally obtained refractive index values of progesterone and estradiol hormones which have been obtained with the help of Atago PAL-RI portable refractometer. In order to get more realistic results, the concentration dependent refractive index values of progesterone and estradiol hormones obtained theoretically with the help of relations 10 and 11 respectively have been synchronized with experimentally obtained refractive index values of progesterone and estradiol hormones. For this purpose cubic curve fitting has been applied on the experimentally obtained discrete refractive index values of progesterone and estradiol hormones to get the relations 10 and 11 respectively. The theoretical cubic curve fitting data along with experimentally obtained data of refractive index values of progesterone and estradiol hormones are plotted in figures 3(a) and (b) respectively.
Figure 3. Theoretically obtained refractive index values of blood samples containing (a) progesterone and (b) estradiol hormones dependent on their concentration levels by applying cubic curve fitting on experimental data.
Download figure:
Standard image High-resolution imageFigures 3(a) and (b) show the refractive index variation of cubic curve fitting and experimental data corresponding to blood sample containing progesterone and estradiol hormones of different concentrations respectively. Refractive index of progesterone hormone varies between 1.3352 to 1.3358 as its concentration level in blood sample changes from 0 to 31 nmol l−1 as shown in figure 3(a). Beside this the refractive index values of estradiol hormone swiftly changes from 1.3332 to 1.3359 as we change concentration level in blood sample from 0 to 1.3 nmol l−1 respectively. Further increase in the concentration level of estradiol hormone from 1.3 nmol l−1 to 3.1 nmol l−1 causes a little change in the refractive index value of estradiol hormone which varies from 1.3355 to 1.3359 as depicted in figure 3(b). From figures 3(a) and (b) it can be clearly seen that the cubic curve fitting data obtained theoretically by using relations 10 and 11 are well in agreement with the experimentally obtained data from portable refractometer Atago PAL-RI.
3.4. Effect of change in concentration of progesterone in blood samples under amorphous and crystalline phases of GST material on the defect mode
The transmission characteristics of 1D photonic biosensor (PQ)5 GDG(PQ)5at normal incidence has been investigated in this section under the influence of amorphous and crystalline phases of GST material. The transfer matrix method has been applied with the help of MATLAB software to get the results of this study. The structural parameters of the proposed biosensor are in accordance with the parameters as defined in section 3.1. To carry out the investigations first blood sample containing progesterone hormones of concentrations 0 nmol l−1, 60 nmol l−1, 80 nmol l−1 and 100 nmol l−1 have been poured one by one into defect layer region D of air. The transmission spectra of 1D photonic bio-sensing structure (PQ)5 GDG(PQ)5 under crystalline and amorphous phases of GST material corresponding to different blood samples containing progesterone hormone of concentrations 0 nmoll−1 , 60 nmol l−1, 80 nmol l−1 and 100 nmol l−1 are being plotted in figures 4 (a) and (b) respectively.
Figure 4. Transmission spectra of 1D photonic biosensor (PQ)5GDG(PQ)5 at normal incidence loaded with blood samples containing progesterone hormones of concentration 0 nmol l−1, 60 nmol l−1, 80 nmol l−1, and 100 nmol l−1 one by one with cavity of thickness dD = 320 nm under (a) crystalline phase and (b) amorphous phase.
Download figure:
Standard image High-resolution imageFigure 4(a) depicts that under crystalline phase of GST material four distinguishable defect modes corresponding to blood samples of concentrations 0 nmol l−1, 60 nmol l−1, 80 nmol l−1 and 100 nmol l−1 are found between 692 nm to 696 nm inside photonic band gap. The reason of these distinguishable defect modes in side PBG is due to minute change in the concentration dependent effective refractive indices of blood samples containing progesterone hormone as described in relation 10. The new location of the defect mode shifted to higher wavelength side is dependent upon the concentration of progesterone hormones in blood sample which varies from 0 nmol l−1 to 100 nmol l−1. The standing wave concept of laser cavity can also be used to understand the presence of defect mode inside PBG as noticed in figure 3. According to standing wave concept of laser cavity only those wavelengths inside cavity can be come out which satisfy the relation δ = kλ = neff L. Here notations δ, k, λ, neff and L have been used to represent optical path difference, an integer, wavelength inside cavity, effective refractive index and cavity length respectively. Moreover the transmittance of the defect modes corresponding to various blood samples of different concentration slightly varies above 90% which is good for any photonic biosensing application. The transformation of GST from aGST to cGST phases under the above mentioned circumstances exhibits significant change in defect mode location inside PBG with moderate reduction in its transmittance as shown in figure 4(b). It also shows that under amorphous phase of GST material defect modes of concentrations 0 nmol l−1, 60 nmol l−1, 80 nmol l−1 and 100 nmol l−1 have repositioned their location between 718 nm to 722 nm. Beside this the concentration dependent transmittance of these defect modes also vary from 70% to 76% which is more than enough for photonic bio-sensing applications. This is due to fact that under crystalline phase KcGST is higher in contrast to KaGST as evident from figure 2 which signifies optical loss. Another observation is that under crystalline phase full width half maximum of defect mode increases which in turn affects the system performance. Thus the application of GST material in fabrication of modern photonic devices can also be used for designing of switchable and reconfigurable photonic biosensors.
3.5. Effect of change in concentration of estradiol hormone in blood sample under amorphous and crystalline phases of GST material on defect mode
Next we have studied how defect mode position and intensity inside PBG will be affected under amorphous and crystalline phases of GST material when cavity is loaded with blood samples containing estradiol hormone of concentration 0 nmol l−1, 6 nmol l−1, 8 nmol l−1 and 10 nmol l−1 one by one. For this reason we have plotted transmission spectra of the proposed bio-sensing design when the cavity is loaded with blood samples containing estradiol hormone of concentration 0 nmol l−1, 6 nmol l−1, 8 nmol l−1 and 10 nmol l−1 under the influence of amorphous and crystalline phases of GST material as shown in figures 5(a) and (b) respectively.
Figure 5. Transmission spectra of 1D photonic biosensor (PQ)5 GDG(PQ)5 at normal incidence loaded with blood samples containing estradiol hormones of concentration 0 nmol l−1, 6 nmol l−1, 8 nmol l−1, and 10 nmol l−1 one by one with cavity of thickness dD = 320 nm under (a) crystalline phase and (b) amorphous phase.
Download figure:
Standard image High-resolution imageUnder crystalline phase of GST material four closely spaced distinguishable defect modes corresponding to blood samples containing estradiol hormones of concentration 0 nmol l−1, 6 nmol l−1, 8 nmol l−1 and 10 nmol l−1 are found between wavelength 690 nm to 710 nm inside PBG of the structure under consideration as presented in figure 5(a). These defect modes shifts toward higher wavelength side as concentration of blood sample increases but their intensity slightly decreases from 98% to 90% as depicted in figure 5(a). The switching of GST material from crystalline phase to amorphous phase results lateral shift in the position of closely spaced distinguishable defect modes inside PBG between wavelength range 710 nm to 735 nm as shown in figure 5(b). Interestingly we have noticed that under amorphous phase the intensity of these defect modes increases from 72% to 89% depending upon the concentration of estradiol hormone in blood sample from 0 nmol l−1 to 10 nmol l−1 contrary to the findings of same blood sample under crystalline phase as shown in figure 5(a). Moreover full width hall maximum of each defect mode under amorphous phase is reduced marginally as compared to the results of figure 5(a). Thus the application of phase change GST materials can be used to improve the performance of reconfigurable photonic bio-sensing devices.
3.6. Effect of variation in defect layer thickness on the performance of the design under cGST and aGST phases of Ge2Sb2Te5 material when the cavity is loaded with progesterone sample of concentration 20 nmol l−1
Further the effect of variation in the thickness of defect layer region loaded with blood sample containing progesterone hormone of concentration 20 nmol l−1, on the performance of the device under cGST and aGST phases of Ge2Sb2Te5 material has been investigated. For this purpose transmission spectra of proposed bio-sensing design at θ0 = 0° with defect layer thicknesses 320 nm, 340 nm, 360 nm and 380 nm under the influence of crystalline and amorphous phases of GST material have been plotted in figures 6(a) and (b) respectively.
Figure 6. Transmission spectra of 1D photonic biosensor (PQ)5GDG(PQ)5 at normal incidence loaded with blood sample containing progesterone hormones of concentration 20 nmol l−1 corresponding to cavity of different thicknesses 320 nm, 340 nm, 360 nm and 380 nm under (a) crystalline phase and (b) amorphous phase.
Download figure:
Standard image High-resolution imageFigure 6(a) depicts four evenly spaced narrow defect modes of intensity more than 95% which are located between wavelength range 690 nm to 720 nm inside PBG of the structure. As the thickness of cavity region increases from 320 nm to 380 nm in steps of 20 nm, position of the narrow defect mode inside PBG evenly shifts toward higher wavelength side between 690 nm to 720 nm inside PBG. Moreover this increase in thickness of cavity region results the variation in intensity from 98% to 95% which is quite good for photonic bio-sensing application. Next we switch GST material from crystalline to amorphous phase and keeping other parameters of the design identical to evaluate the performance of the design under the influence of amorphous phase of GST material. The transmission spectra of proposed design loaded with blood sample containing progesterone hormone of concentration 20 nmol l−1 corresponding to defect layer thicknesses 320 nm, 340 nm, 360 nm and 380 nm under amorphous phase has been plotted in figure 6(b). It shows that under the influence of amorphous phase of GST material, PBG is almost stagnant to that of figure 6(a). On the other hand defect mode positions corresponding thicknesses 320 nm, 340 nm, 360 nm and 380 nm are shifted to new positions inside PBG and are located between wavelength range 712 nm to 750 nm as shown in figure 6(b). The increase in the thickness of defect layer region results corresponding increase in its intensity which varies from 74% to 100% corresponding to thickness variation from 320 nm to 380 nm respectively. Beside this the increase in the thickness also increases the full width half maximum of defect mode inside PBG which in turn alters the performance of the design.
3.7. Effect of variation in defect layer thickness on the performance of the design under cGST and aGST phases of Ge2Sb2Te5 material when the cavity is loaded with estradiol sample of concentration 7 nmol l−1
In this section we have estimated the performance of the proposed photonic biosensor by changing the thickness of cavity region from 320 nm to 380 nm insteps of 20 nm when the cavity is loaded with blood sample containing estradiol hormone of concentration 7 nmol l−1 under crystalline and amorphous phases of GST material. For this purpose we have plotted transmission spectra corresponding to crystalline and amorphous phases of GST material as shown in figures 7(a) and (b) respectively.
Figure 7. Transmission spectra of 1D photonic biosensor (PQ)5GDG(PQ)5 at normal incidence loaded with blood sample containing estradiol hormones of concentration 7 nmol l−1 corresponding to cavity of different thicknesses 320 nm, 340 nm, 360 nm and 380 nm under (a) crystalline phase and (b) amorphous phase.
Download figure:
Standard image High-resolution imageFigure 7(a) shows evenly spaced distinguishable defect modes of transmittance which varies from 98% to 92% corresponding to thickness variation from 320 nm to 380 nm respectively under crystalline phase of GST material. These defect modes are located between wavelength range 690 nm to 718.5 nm inside PBG and have almost identical full width half maximum. Further we switch Ge2Sb2Te2 material from cGST to aGST phases keeping other parameters identical and observe that the effect of this change on the performance of the device in comparison to performance under crystalline phase as shown in figure 7(a). The transmission spectra showing performance of the design under amorphous phase of GST corresponding to defect layer thicknesses 320 nm, 340 nm, 360 nm and 380 nm, has been plotted in figure 7(b) at normal incidence. It shows that under amorphous phase of GST, position of PBG is almost stagnant but the defect modes inside this PBG are repositioned to new locations between wavelength range 720 nm to 750 nm. The intensity of these defect modes gradually increases from 74% to 100% as thickness of defect layer increases from 320 nm to 380 nm respectively. Besides this their FWHM also increases as evident from figure 7(b). Thus the performance of the photonic biosensor is almost identical when the cavity region is loaded separately with blood samples containing progesterone and estradiol reproductive hormones of certain concentration under crystalline and amorphous phase of GST material.
3.8. Effect of change in angle of incidence corresponding to TE polarized electromagnetic wave on the performance of the design when the cavity of thickness 320 nm is loaded with progesterone sample of concentration 20 nmol l−1 under crystalline and amorphous phases of GST material
In this section the effect of change in angle of incidence from 0° to 30° in steps of 10° corresponding to TE polarized electromagnetic wave on the performance of the proposed biosensor has been studied. For this purpose we have loaded the cavity region of thickness 320 nm with blood sample containing progesterone hormone of concentration 20 nmol l−1.
Figures 8(a) and (b) show the transmittance spectra of proposed structure under crystalline and amorphous phase of GST material at normal incidence respectively. Under crystalline phase of GST material figure 8(a) shows that the increase in angle of incidence from 0° to 30° results the shifting of defect modes towards lower wavelength side between wavelength range 694 nm to 670 nm inside the PBG. The gradual increase in the incident angle also decreases the intensity of the defect mode which varies between 94% to 89. The increase in the angle of incidence corresponding to TE polarized waves improves the geometrical path travelled by the light wave inside defect layer loaded with blood sample containing women reproductive hormones. This improvement in the geometrical path ensures much better interaction between light wave and blood sample which in turn improves the performance of the proposed bio-sensing design. Next we switch Ge2Sb2Te5 material from cGST phase to aGST phase keeping all other structural parameters constant. Under these circumstances the transmission spectra has been plotted in figure 8(b). This switching between phases results change in the positions of angle of incident dependent defect modes inside PBG as shown in figure 8(b). Under amorphous phase of GST the intensity of defect modes inside PBG is nearly 72%. This value of intensity is enough for bio-sensing applications. Thus the variation in angle of incidence can also be used to improve the performance as well as conformation of investigations carried by proposed design under cGST and aGST phases of Ge2Sb2Te5 material which induces the reconfigurable property into our proposed photonic bio-sensing design.
Figure 8. Transmission spectra of 1D photonic biosensor (PQ)5GDG(PQ)5 at normal incidence loaded with blood sample containing progesterone hormones of concentration 20 nmol l−1 corresponding to cavity of thickness 320 nm at different incident angles 0°, 10°, 20° and 30° under (a) crystalline phase and (b) amorphous phase.
Download figure:
Standard image High-resolution image3.8.1. Effect of change in angle of incidence corresponding to TE polarized electromagnetic wave on the performance of the design when the cavity of thickness 320 nm is loaded with estradiol sample of concentration 7 nmol l−1 under cGST and aGST phases of Ge2Sb2Te5 material
Here the effect of change in angle of incidence from 0° to 30° in steps of 10° corresponding to TE polarized electromagnetic wave on the performance of the proposed biosensor loaded with blood sample containing estradiol hormone of concentration 7 nmol l−1 has been studied. For this purpose we have fixed the defect layer thickness to 320 nm. Figures 9(a) and (b) show the transmittance spectra of proposed structure under crystalline and amorphous phases of GST material at normal incidence respectively.
Figure 9. Transmission spectra of 1D photonic biosensor (PQ)5GDG(PQ)5 at normal incidence loaded with blood sample containing estradiol hormones of concentration 20 nmol l−1 corresponding to cavity of thickness 320 nm at different incident angles 0°, 10°, 20° and 30° under (a) crystalline phase and (b) amorphous phase.
Download figure:
Standard image High-resolution imageUnder crystalline phase of GST material figure 8(a) shows four defect modes of decreasing intensity corresponding to angle of incidence 0°, 10°, 20° and 30°. These defect modes are located between wavelength range 660 nm to 700 nm inside PBG under cGST phase of Ge2Sb2Te5 material. On the other hand under amorphous phase of GST material the positions of these angle dependent defect modes inside PBG relocate their positions between wavelength range 700 nm to 730 nm. The intensity of these defect modes is almost stagnant which is above 70%. Thus we have similar findings of the proposed design loaded with two different blood samples containing female reproductive hormones under the influence of cGST and aGST phases of Ge2Sb2Te5 material.
3.8.2. Performance evaluation of the proposed design
The evaluation of the performance and efficiency of the proposed design has been done by calculating sensitivity (S), quality factor (Q) and figure of merit (FOM) values because these are most common parameters to evaluate the performance of any biosensor [6, 13–15]. The sensitivity is the ratio of change in the central wavelength of defect mode (Δλ) due to change in the refractive index of cavity region (Δn) as defined below

For getting accuracy in biosensor measurements the quality factor of the biosensor should be as high as possible and it can be calculated with the help of following relation

where λpeak and FWHM are used to represent central wavelength and full width half maximum of transmission peak inside cavity region.
The figure of merit (FOM) of biosensor design is defined as the ratio of sensitivity to FWHM of defect mode inside PBG. FOM can be obtained by using following relation

The evaluation of the performance of the proposed 1D photonic biosensor (PQ)5 GDG(PQ)5 loaded with blood samples containing progesterone and estradiol reproductive hormones of different concentration under crystalline and amorphous phases of GST has been done by calculating S, Q and FOM values of designs depending upon change in internal and external parameters of the design. The numerical values of S, Q and FOM of design under crystalline and amorphous phases have been obtained by using relations (12) to (14) and are listed in table 1 to table 8. Tables 1–4 are summarizing the calculated results of the design corresponding to blood sample with progesterone hormone of concentration 0, 60 nmol l−1, 80 nmol l−1 and 100 nmol l−1 at incident angle 0°and defect layer thickness 320 nm and 380 nm under crystalline and amorphous phases of GST. On the other hand tables 5–8 are summarizing above mentioned findings for blood sample containing estradiol hormone of concentration 0, 6 nmol l−1, 8 nmol l−1 and 10 nmol l−1 under crystalline and amorphous phases of GST. The comparison between the results of table 1 and table 2 yields that under given circumstances with progesterone sample at dD = 320 nm the performance of the proposed design in terms S, Q and FOM values can be improved significantly by switching amorphous to crystalline phase of GST material. The similar findings have been worked out with dD = 380 nm as per data mentioned in tables 3 and 4 corresponding to crystalline and amorphous phases respectively. We have also evaluated the performance of the devise when cavity is loaded with blood samples containing estradiol hormones of different concentration at dD = 320 nm and 380 nm under amorphous and crystalline phases of GST material. The results associated with these findings are presented in tables 5–8. Additionally the order of limit of detection of our design is 10–5. It helps to estimate the smallest change in the refractive index of analyte which could be significantly detected by proposed structure. Thus we can conclude that GST can be a better choice for designing 1D defective photonic structures suitable for refractive index based sensing applications. Moreover the GST based photonic devices are reconfigurable and externally tunable.
Table 1. The performance of proposed design (PQ)5
GDG(PQ)5 loaded with blood sample containing progesterone hormones of different concentration with at θ0 = 0° under crystalline phase of GST.
Types of hormones | C (nmol l−1) | Refractive index |
![]() |
![]() | S (nm/RIU) |
![]() | FOM (RIU) |
---|---|---|---|---|---|---|---|
Progesterone | 0 | 1.33520 | 718.33 | 0.05 | — | 14367 | — |
60 | 1.33899 | 718.85 | 0.05 | 137.02 | 14377 | 2740.5 | |
80 | 1.34621 | 719.54 | 0.06 | 62.67 | 11992 | 1044.5 | |
100 | 1.35983 | 721.00 | 0.05 | 59.28 | 14420 | 1185.6 |
Table 2. The performance of proposed design (PQ)5
GDG(PQ)5 loaded with blood sample containing progesterone hormones of different concentration with at θ0 = 0° under amorphous phase of GST.
Types of hormones | C (nmol l−1) | Refractive index |
![]() |
![]() | S (nm/RIU) |
![]() | FOM (RIU) |
---|---|---|---|---|---|---|---|
Progesterone | 0 | 1.33520 | 692.83 | 0.06 | — | 11547 | — |
60 | 1.33899 | 693.14 | 0.06 | 81.69 | 11552 | 1361.5 | |
80 | 1.34621 | 693.77 | 0.06 | 57.22 | 11563 | 953.7 | |
100 | 1.35983 | 694.97 | 0.06 | 48.72 | 11583 | 812.0 |
Table 3. The performance of proposed design (PQ)5
GDG(PQ)5 loaded with blood sample containing progesterone hormones of different concentration with at θ0 = 0° under crystalline phase of GST.
Types of hormones | C (nmol l−1) | Refractive index |
![]() |
![]() | S (nm/RIU) |
![]() | FOM (RIU) |
---|---|---|---|---|---|---|---|
Progesterone | 0 | 1.33520 | 715.98 | 0.06 | — | 11933 | — |
60 | 1.33899 | 716.40 | 0.06 | 110.67 | 11940 | 1844.7 | |
80 | 1.34621 | 717.14 | 0.05 | 67.212 | 14342.8 | 1344.2 | |
100 | 1.35983 | 718.61 | 0.05 | 59.68 | 14372.2 | 1193.7 |
Table 4. The performance of proposed design (PQ)5
GDG(PQ)5 loaded with blood sample containing progesterone hormones of different concentration with at θ0 = 0° under amorphous phase of GST.
Types of hormones | C (nmol l−1) | Refractive index |
![]() |
![]() | S (nm/RIU) |
![]() | FOM (RIU) |
---|---|---|---|---|---|---|---|
Progesterone | 0 | 1.33520 | 743.16 | 0.2 | — | 3715.8 | — |
60 | 1.33899 | 743.37 | 0.21 | 55.33 | 3639.9 | 263.5 | |
80 | 1.34621 | 743.85 | 0.26 | 43.59 | 2860.9 | 167.7 | |
100 | 1.35983 | 744.625 | 0.31 | 31.50 | 2402.0 | 101.6 |
Table 5. The performance of proposed design (PQ)5
GDG(PQ)5 loaded with blood sample containing estradiol hormones of different concentration with at θ0 = 0° under crystalline phase of GST.
Types of hormones | C (nmol l−1) | Refractive index |
![]() |
![]() | S (nm/RIU) |
![]() | FOM (RIU) |
---|---|---|---|---|---|---|---|
Estradiol | 0 | 1.33330 | 692.65 | 0.06 | — | 11544.2 | — |
6 | 1.34997 | 694.11 | 0.05 | 87.85 | 13882.2 | 1757.04 | |
8 | 1.38723 | 697.41 | 0.05 | 61.194 | 13948.2 | 1223.88 | |
10 | 1.46175 | 704.20 | 0.05 | 52.86 | 14084.0 | 1057.23 |
Table 6. The performance of proposed design (PQ)5
GDG(PQ)5 loaded with blood sample containing estradiol hormones of different concentration with at θ0 = 0° under amorphous phase of GST.
Types of hormones | C (nmol l−1) | Refractive index |
![]() |
![]() | S (nm/RIU) |
![]() | FOM (RIU) |
---|---|---|---|---|---|---|---|
Estradiol | 0 | 1.33330 | 718.11 | 0.07 | — | 10258.7 | — |
6 | 1.34997 | 719.96 | 0.1 | 110.98 | 7199.6 | 1109.78 | |
8 | 1.38723 | 723.9 | 0.1 | 73.06 | 7239.0 | 730.62 | |
10 | 1.46175 | 731.01 | 0.11 | 55.35 | 6645.6 | 503.21 |
Table 7. The performance of proposed design (PQ)5
GDG(PQ)5 loaded with blood sample containing estradiol hormones of different concentration with at θ0 = 0° under crystalline phase of GST.
Types of hormones | C (nmol l−1) | Refractive index |
![]() |
![]() | S (nm/RIU) |
![]() | FOM (RIU) |
---|---|---|---|---|---|---|---|
Estradiol | 0 | 1.33330 | 715.8 | 0.06 | — | 11930.0 | — |
6 | 1.34997 | 717.55 | 0.06 | 104.98 | 11959.7 | 1749.65 | |
8 | 1.38723 | 721.49 | 0.09 | 73.062 | 8016.6 | 811.80 | |
10 | 1.46175 | 729.12 | 0.11 | 59.40 | 6628.4 | 540.01 |
Table 8. The performance of proposed design (PQ)5
GDG(PQ)5 loaded with blood sample containing estradiol hormones of different concentration with at θ0 = 0° under amorphous phase of GST.
Types of hormones | C (nmol l−1) | Refractive index |
![]() |
![]() | S (nm/RIU) |
![]() | FOM (RIU) |
---|---|---|---|---|---|---|---|
Estradiol | 0 | 1.33330 | 743.07 | 0.28 | — | 2653.8 | — |
6 | 1.34997 | 744.2 | 0.31 | 67.79 | 2400.7 | 218.67 | |
8 | 1.38723 | 746.46 | 0.39 | 41.91 | 1914.0 | 107.46 | |
10 | 1.46175 | 750.37 | 0.58 | 30.44 | 1293.7 | 52.48 |
Finally we have compared our results with some excellent piece of research work carried by distinguished photonic workers in table 9. This comparison highlights the usefulness of our proposed design in contrast to the previous research works on refractive index based sensing mechanism having smaller sensitivity. The sensitivity of our structure can be easily tailored conveniently by changing incident angle as well as thickness of defect layer region. Beside this sensitivity can also be tailored by switching states of Ge2Sb2Te5 material from aGST to cGST phases and back. The presence of GST material does not require phase matching conditions similar to the devices based on surface plasmon resonance (SPR) to achieve tunable sensitivity. The order of quality factor and figure of merit values of the proposed photonic biosensor are similar to majority of schemes cited below in table 9. The experimental realization of the proposed design based on refractive index sensing technology has been shown in figure 1. Here we required a wide band source of light through which light can be launched into proposed structure with the help of single mode fiber and directional couplers. The blood sample flow chamber should be mounted near the cavity layer with IN and OUT facility for sample to be poured. The interaction between light and blood sample under investigation results the change in position of defect mode inside PBG. This shifting of defect mode indie PBG of transmission spectra is analyzed with the help of optical spectrum analyzer and final results will be displayed in computer with the help of software. It is evident from table 9 that the proposed biosensor possesses relatively good sensitivity and much better performance to detect reproductive hormones in females in contrast to the resent bio-sensing research work.
Table 9. Comparison of numeric values of sensitivity, quality factor and figure of merit of proposed design with previous research work of similar kind for evaluating the performance of the proposed bio-sensing design (PQ)5GDG(PQ)5.
Year | S (nm/RIU) | Q-factor | FOM (RIU) | Frequency Range | References |
---|---|---|---|---|---|
2016 | 34.11 | Not mentioned | 1.1 × 103 | THz | [38] |
2017 | 17 | 3 × 104 | 2.23 × 102 | Visible to NIR | [39] |
2019 | 25.75–51.49 | Not mentioned | Not mentioned | NIR | [40] |
2019 | 32–43.13 | Not mentioned | Not mentioned | NIR | [41] |
2019 | 53.0–90.9 | Not mentioned | Not mentioned | NIR | [42] |
2020 | 10 | 3 × 102 | 15.1 | Visible | [43] |
2021 | 71–75 | Not mentioned | Not mentioned | NIR | [44] |
This work | 137.02–30.44 | (0.12–1.43) × 104 | (0.2–2.7) × 103 | Visible | ... |
4. Conclusion
In conclusion we have studied the effect of GST material which has been used either side of cavity region as a buffer layer to make our biosensor reconfigurable with ability of bistable active tuning of defect mode inside photonic band gap of 1D photonic bio-sensing structure (PQ)5GDG(PQ)5. These special features are induced in our design due to the different optical response of GST material under crystalline and amorphous phases. We have investigated blood samples containing reproductive hormones of different concentration to evaluate the performance of the proposed biosensor. The transfer matrix method and MATLAB software both have been utilized to get transmission properties of the 1D photonic bio-sensing structure air/(PQ)5GDG(PQ)5/air. The proposed photonic structure shows high sensitivities of 137 nm R−1IU−1 and 81.69 nm R−1IU−1 when the cavity of thickness 320 nm is loaded with progesterone hormone of concentration 60 nmol/L under crystalline and amorphous phases respectively of GST material. On the other hand corresponding to estradiol hormone of concentration 6 nmol l−1 the sensitivity of the device has been found to 87.85 nm RIU and 110.98 nm/RIU under crystalline and amorphous phases of GST respectively. Apart from sensitivity other parameters like quality factor and figure of merit have also been studied to get deep insight about the sensing capabilities of the proposed design under the influence of crystalline and amorphous phases of GST. These findings may pave path for design and development of various reconfigurable photonic sensing devices based on GST. The proposed design may also be used to configure level free bio-sensing devices to determine gynecological problems pertaining to reproductive hormones in females.
Data availability statement
All data that support the findings of this study are included within the article (and any supplementary files).
Funding
This research received no external funding.
Conflicts of interest
The authors declare that there are no conflicts of interest.