Abstract
Smartphone-based experimental exercises were incorporated as part of the homework problems in an introductory mechanics course at a university. A quasi-experimental field study with two cohorts design was performed to measure the impact of such exercises on motivation, interest and conceptual understanding. The empirical results on learning achievement show a significant positive influence of the smartphone-based experimental exercise for the dynamics of rigid bodies topic with a medium effect size of d = 0.42. For the analysis of rotational motion topic, a positive learning achievement for both groups was evidenced, but the effect size of the smartphone-based exercise was rather small at d = 0.20 . The intrinsic and germane cognitive loads turned out to be similar at an intermediate level for both groups. However, the extrinsic cognitive load for the intervention group decreased significantly, which might be the reason why more complex experimental exercises foster conceptual understanding.
Export citation and abstract BibTeX RIS

Original content from this work may be used under the terms of the Creative Commons Attribution 4.0 license. Any further distribution of this work must maintain attribution to the author(s) and the title of the work, journal citation and DOI.
1. Introduction
The use of smartphones as digital measurement and experimentation media in physics has become part of modern physics teaching and learning. Via suitable exercises, smartphones with their internal sensors and special apps offer the opportunity for students to explore physical phenomena under their own control and speed [1–6]. This expansion of the physical problem repertoire in school and university physics classes is shown to be accompanied by achievements in the motivational and cognitive learning successes of the learners, which are predominantly discussed positively in the literature [7–10]. Nonetheless, the research situation on this topic remains weak. Hillmayr et al [9] reported that digital media have a medium effect (Hake index g = 0.62) on learning performance. These general statements can also be applied to the use of smartphones. Mazella and Testa [10] showed that smartphone-based assignments can be a valuable substitute for traditional teaching–learning settings for the concept of acceleration. Hochberg et al [8] showed that for approximately 50 students of a German secondary school (12th form), the use of smartphones and tablets led to a significantly higher learning achievement compared to a traditional learning setting.
Our own research with respect to the incorporation of smartphone-based experiments for physics education at universities focuses on specially designed experimental exercises, in which students undertake as bi-weekly homework. For this so-called smart physics lab (SPL), we adapted experimental ideas for smartphones from the literature and designed a number of attractive and challenging tasks on our own, such as e.g. the analysis of kinematic data from GPS recordings of a free motion [11], the measurement of the moment of inertia of the tilting smartphone [12] and the determination of the shear modulus of a thin wire via a torsion pendulum, were the smartphone serves as pendulum bob and measurement device for the oscillating angular velocity [13]. The principles of how these experiments may be implemented in physics courses at university were described in a previous paper in this journal [14]. The reader is also referred to several papers from colleagues of the phyphox development team at RWTH Aachen, who also apply smartphone experiments in university physics education [1, 2, 15].
The present paper presents the results of a follow-up study, in which we measure the contribution to the learning achievement due to smartphone-based experimental exercises replacing partially traditional paper–pencil tests for students in the first semester of their physics studies at universities. The study is designed as a quasi-experimental field study with an intervention group (IG) using smartphone-based exercises and a control group (CG) working with traditional paper–pencil tests. Additionally, we investigated the influence of the exercise type on curiosity, motivation and cognitive load of the students and put the results in context with the measured learning achievement for two different topics in the area of mechanics.
2. The SPL
Since the winter term 2018/2019, the SPL has been an integral part of the introductory mechanics course for physics teacher trainees at Leipzig University. The aim is to improve students' understanding of physics concepts and to strengthen their motivation as well as their interest in physics in the introductory phase of their studies. The smartphone serves as a universal physical measurement device that can be used in adapted experimental tasks. By working with their own smartphone or tablet, a new and comprehensive approach to the ways of thinking and working in physics is offered. Students go through the steps from theoretically presented models in lectures and textbooks to real models observed and digitally recorded with their own smartphones. Using this problem-solving process, students identify the conditions under which the physical models fit real-world problems.
Figure 1 shows the integration of the SPL during the winter term 2021/2022. As in the two previous winter terms, it was part of the introductory mechanics course during the first semester, which consisted of min lectures and
min recitation every week. In order to be admitted to the exam, students received weekly homework, 50% of which must be solved correctly. Some of these tasks were replaced by smartphone-based experimental exercises. The students were allowed 2 weeks processing time to complete these experimental exercises and were required to hand in short reports (protocols) of the experiment they performed.
Figure 1. The smart physics lab in the winter term 2021/2022 with five integrated smartphone-based exercises SPL01–SPL05. The exercises highlighted in red are part of the learning effectiveness study.
Download figure:
Standard image High-resolution imageAt the beginning of our mechanics course, students received an introduction on how to successfully use smartphones for quantitative experiments in physics. This introduction was presented in a designated expert lecture and during the first recitation. During the lecture, we presented the centrifugal acceleration experiment of the phyphox team as a demonstration experiment [15]. In order to support the students, an accompanying tutorial was provided. Here, the students practiced experimentation with their smartphones and discussed their own approaches to solve the experimental exercise. In cases where the smartphone of the students might not be equipped with the necessary sensors to solve a specific experimental task, we lend suitable smartphones and tablets to the students upon request.
3. Methods
3.1. Research design
This study was pre-piloted and tested with the SPL04 tilting smartphone exercise (see figure 1) during the winter term 2020/2021. A quasi-experimental field study with a CG and IG setup with pre- and post-tests to measure the learning achievement was chosen. The selection and composition of the control and intervention groups as well as the study design proved to be suitable. The results of the pre- and post-tests showed a positive influence on the learning achievement for the experimental exercise. Based on the results of the pilot phase, the pre- and post-test contents were revised in order to improve their validity and reliability. In addition, the scales for measuring interest and motivation via surveys in the beginning and at the end of the intervention were changed from a six-point to a four-point Likert scale for the follow-up study [16].
The presented study took place during the winter term 2021/2022 with first-semester students on the experimental physics 1 course (mechanics). It was designed to be similar to the pilot phase and was carried out for two successive experimental exercises, SPL03 and SPL04, highlighted in red in figure 1. A total of 85 students participated in all pre- and post-tests. The IG consisted of the first semester students enrolled in our physics teacher trainee courses. The CG consisted of the first semester bachelor of science physics and bachelor of science meteorology students.
The timeline of the study is shown in figure 2. At the beginning (τ0), the demographic data of the two cohorts were collected via a self-report. During the same survey, a questionnaire was used to measure the motivation and interest of the students at the beginning of their study via a four-point Likert scale. The measurement of these two variables was based on existing, valid test instruments [17, 18].
Figure 2. Design of the study as a quasi-experimental field study with control and intervention groups in the winter term 2021/2022.
Download figure:
Standard image High-resolution imageThe topics investigated during the two intervention phases by the IG were the analysis of circular motions during the interval with the SPL03 and the dynamics of rigid bodies during the interval
with the SPL04. For each topic, paper–pencil tests were developed in which students in the CG had to apply and link the same physical concepts as part of their weekly homework.
Figures 3(a) and (b) show sketches and measurement data of both interventions. In the IG (task SPL03), students were asked to determine the position of the micro-electromechanical systems (MEMS) accelerometer within their smartphone by rotating it in two different orientations. They were required to measure the centrifugal acceleration dependence on the angular velocity and determine the distance of the MEMS from the axis of rotation. Finally, a coordinate transformation to the frame of reference associated with the edges of the smartphone yielded the required coordinates. In a sample solution (see figure 3(a)), the smartphone was mounted on a door leaf in two different orientations where it was allowed to move on a circular path with a fixed axis of rotation. In the IG, SPL04 students analysed the tilting motion of their smartphone. Using the conservation of energy and Steiner's theorem, students determined the moment of inertia of their smartphone from the maximum of the angular velocity measured with the internal gyroscope just before it met a horizontal soft mat (see figure 3(b)). They were required to compare the experimental results for the moment of inertia with the values calculated under the assumption that the smartphone is a homogenous cuboid.
Figure 3. Sketch and example measurement data for both interventions SPL03 (a) and SPL04 (b).
Download figure:
Standard image High-resolution imageThe main difference between the smartphone-based experimental exercise and the pencil–paper test of the CG was that the students in the IG performed the experiment with their own smartphone and used self-generated data to evaluate physical models, whereas for the students in the CG, independent experimentation was omitted and the solution needed to be derived via a calculus-like algorithm in the cookbook principle. The exercise text for both groups can be found in the supplementary material (available online at stacks.iop.org/PhysEd/57/045038/mmedia).
3.2. Measured variables
Before the students received their respective exercise sheets, pre-tests were conducted to measure the prior knowledge level of both groups regarding each topic (see figure 2). After the experimental and the paper–pencil homework were submitted by the students, post-tests
were given to both groups to measure the learning achievement for each of the two subject areas. Each test consisted of nine single-choice questions for intervention 1 (analysis of circular motion) and of 10 single-choice questions for intervention 2 (dynamics of rigid bodies). The concept questions of the pre- and post-tests were derived from validated test instruments [19–21] and adapted from questions from textbooks [22–24], respectively. Our own developments of concept questions were validated during the pre-pilot phase.
At the end of the study , a final survey was conducted to measure changes in student motivation and interest again on a four-point Likert scale. In addition, questions were asked about the cognitive load of the respective exercises via a six-point Likert scale [25]. The cognitive load test instrument used allowed us to distinguish between three types of cognitive load [25]. The intrinsic cognitive load results from the exercise difficulty, its complexity, and its requirements in terms of time and content. The extraneous cognitive load depends on the didactic design of the task. It describes the load on the working memory that is irrelevant to learning and influences the structuring of the knowledge transfer and the presentation of the learning content. If it is too high, there are too few cognitive resources available to achieve the learning content. With the germane cognitive load, the free cognitive resources are designated, which can be raised for real knowledge construction in the working memory.
For measurement of the learning achievement between pre- and post-tests, the Hake index g was determined [26]. It is calculated from the percentages of correct answers in the pre-test ppre and the post-test . The Hake index describes the ratio between the reached learning achievement
and the maximum possible learning achievement
. For evaluation of the data collected via the surveys and the concept tests, covariance analyses including the factor group membership or t-tests were utilized.
4. Results and discussion
4.1. Demographic data
A summary of demographic data for the IG and CG is presented in table 1. With more than 70%, the majority of the members in both groups are less than 22 years old. Thus, both groups consist mainly of students participating for the first time in a university course of experimental physics. With respect to their secondary school education in mathematics and physics, i.e. the participation in advanced courses and their averaged A-level degrees received, there are also no significant differences between the IG and the CG. This is indicated by the p values in table 1, which were obtained via a t-test comparing both groups and which are all found to be p > 0.05. In summary, the CG and IG are similar with respect to composition and to performance of their members in mathematics and physics from secondary school education.
Table 1. Demographic data for the intervention and control group. The last four lines refer to the courses taken and A-level degrees achieved.
IG | CG | Significance p | |
---|---|---|---|
Number of participants | 40 | 45 | — |
Female students | 15 | 25 | — |
![]() | 71% | 75% | — |
Advanced course mathematics | 80% | 73% | 0.08 |
Mathematics A-level degree |
![]() |
![]() | 0.56 |
Advanced course physics | 55% | 54% | 0.41 |
Physics A-level degree |
![]() |
![]() | 0.11 |
4.2. Curiosity and motivation
Table 2 provides an overview of the number of items for each of these variables and their reliability. The reliability (Cronbach's α) of the scales is always . Thus, the items and the scales applied in this survey can be considered acceptable [27]. By analysing the variables of motivation and curiosity prior to the first intervention (τ0), no systematic group differences were found (see τ5 table 2). In both groups, motivation and interest in studying physics remained at approximately the same intermediate level during the post-intervention survey at time τ5. There are several approaches to explain this. The students in the IG have been working with the smartphone-based tasks since the beginning of the semester. Thus, the novelty effect, which often increases curiosity and motivation, partially expires by the time of the intervention. In addition, our experimental exercises are at a higher academic level compared to similar studies [7, 8]. Thus, the difficulty level and the pressure situation of the required exam admission might have limited a considerable increase in motivation and curiosity.
Table 2. Development of motivation and curiosity over the course of the study. Average and standard deviation σ from the four-point Likert scale survey as well as the t-values and significances p of the statistical analysis of the data for both groups. The applied scale ranges from 1 (high) to 4 (small).
IG | CG | ||||||||
---|---|---|---|---|---|---|---|---|---|
Time | Items |
![]() | σ |
![]() | σ | t | p | α | |
Curiosity | τ0 | 7 | 2.15 | 0.81 | 2.11 | 0.84 | 0.24 | 0.42 | 0.77 |
τ5 | 8 | 1.97 | 0.68 | 1.82 | 0.69 | 0.97 | 0.16 | 0.75 | |
Motivation | τ0 | 6 | 2.09 | 0.72 | 1.99 | 0.68 | 0.63 | 0.26 | 0.73 |
τ5 | 7 | 2.25 | 0.74 | 2.09 | 0.73 | 0.97 | 0.17 | 0.70 |
4.3. Cognitive load
Table 3 shows the comparison of average and standard deviations of the Likert-scale data for the three types of cognitive load for both groups after successful completion of all tasks in this study (τ5). The reliability of the scale of the seven test items used is with α = 0.78 also acceptable [27]. It can be seen that the intrinsic and the germane cognitive load do not differ for the two groups. However, the extrinsic cognitive load differs significantly for both groups (see p < 0.05 in table 3, line 4). The CG value of 3.1 is lower than that of the IG of 3.61.
Table 3. Comparison of the three types of cognitive load. Average and standard deviation σ from the six-point Likert scale survey, as well as the t-value and significance p of the statistical analysis of the data of both groups. The applied scale ranges from 1 (high) to 6 (small).
IG | CG | ||||||
---|---|---|---|---|---|---|---|
Items |
![]() | σ |
![]() | σ | t | p | |
Intrinsic load | 2 | 3.06 | 0.94 | 3.18 | 1.03 | 0.55 | 0.29 |
Extrinsic load | 3 | 3.61 | 1.33 | 3.12 | 1.13 | 1.80 | 0.04 |
Germane load | 2 | 2.03 | 0.56 | 2.01 | 0.73 | 0.13 | 0.45 |
The comparison of intrinsic and germane cognitive loads for both groups after the two interventions shows that the smartphone-based exercises are as demanding for the IG as the traditional paper–pencil tests are for the CG. This means that the task-based measurement and analysis process does not impose such additional loads on the IG learners. For the IG, the extrinsic cognitive load is lower than for the CG with the paper–pencil tests. Obviously, the experimental exercises allow a more elementary access to the physical concepts of the respective topics than the traditional pencil–paper tests. Thus, learners benefit from the use of smartphone-based exercises and can be more conducive to learning.
4.4. Learning achievement
Figure 4 compares the proportions of correct answers for both groups in the pre- and post-tests for both interventions. For the analysis on circular motion topic during , 64% correct answers were given in the pre-test of the IG. At 54%, the figure is lower for the CG. In the post-test, the probability of a correct answer increases to 71% for the IG and to 59% for the CG, respectively. Obviously, both groups were able to increase their performance. However, the CG achieved a slightly greater increase in learning than the IG.
Figure 4. Comparison of the pre- and post-test results for both interventions SPL03 and SPL04 for the control group (red) and the intervention group (blue).
Download figure:
Standard image High-resolution imageThe proportions of correct answers for both groups in the pre- and post-test for the intervention the dynamics of rigid bodies topic during shows that the IG was able to increase its proportion of correct answers from 72% in the pre-test to 76% in the post-test. The CG was also able to improve its results, but the increase here was only from 61% to 64%.
To investigate the learning achievement, the Hake index gi
was calculated for each participant in the study from data from the pre-test and the post-test. Table 4 presents the averaged learning achievement for both groups and topics. For the IG, the learning achievement is larger than for the CG in both interventions. The calculated learning achievements were additionally examined by means of covariance analysis (p < 0.05). The only factor taken into account was group membership. In addition, Cohen's d was calculated as an effect size measure. It was found that for the dynamics of ridged bodies topic the learning achievement of the IG working with the smartphone-based exercises is significantly higher compared to the achievement of the CG solving a traditional paper–pencil test (see table 4). A medium effect size of d = 0.42 was calculated. For the analysis of rotational motion topic, there is, however, no evidence for a significant increase of a higher learning achievement for the IG compared to the CG. The effect size for this intervention is with a Cohen's d of d = 0.20 considered to be small.
Table 4. Comparison of the learning achievement for both groups and both exercises. The significance p and the effect size Cohen's d of a covariance analysis are also given.
IG | CG | |||
---|---|---|---|---|
![]() |
![]() | d | p | |
SPL 03: analysis of rotational motion | 0.19 | 0.11 | 0.20 | 0.37 |
SPL 04: dynamics of rigid bodies | 0.28 | 0.08 | 0.42 | 0.04 |
These data agree with Mazella and Testa's finding [10] that smartphone-based exercises are an attractive alternative to the traditional paper–pencil tests. The main difference between the compared exercises is that the students in the IG use self-generated experimental data and evaluate them accordingly, while the students in the CG do not have to experiment themselves. The close theory–experiment interplay supports the knowledge transfer for the IG. The learning results show a positive trend in favour of the presented smartphone-based exercises. For physics courses at universities, they confirm the results of Hillmayr et al [9] and Hochberg et al [7] that smartphone-based exercises have a positive effect on physics learning.
The effect size of our smartphone-based exercises strongly depends on the topic and the task itself. The analysis of rotational motion topic (first intervention) is already discussed during advanced courses in secondary school. Thus, in contrast to the dynamics of rigid bodies topic (second intervention) it is not completely new for the learners on our courses. The experimental problems for the IG and the paper–pencil test for the CG are perhaps too similar in the first intervention and the IG learners were not triggered enough. Thus, careful task and exercise design also remain a challenging task for smartphone-based experimental exercises.
5. Conclusion
We incorporated smartphone-based experimental exercises into our introductory mechanics course at a university. Via a quasi-experimental field study with two cohorts design with pre- and post-tests, this new exercise format was compared with traditional paper–pencil tests. Both exercise types were given to the students of the respective cohorts as homework and addressed the two topics of analysis of rotational motion and dynamics of rigid bodies.
For our students, both exercise types do not exhibit significantly different influences on curiosity and motivation to study physics. The cognitive load, which might be expected to be higher for the smartphone-based experimental exercises, proved to be similar for the intrinsic and the germane fractions of cognitive load. Surprisingly, the extrinsic cognitive load was significantly smaller for the smartphone-based exercise. We assume that it is this decreased extrinsic cognitive load for the smartphone-based exercise that forms the reason for the improved learning achievement measured via the Hake index for the dynamics of rigid bodies topic. Students who are acquainted with using smartphones as measurement devices in physical experiments thus learn more effectively about new topics in the mechanics course than students who receive only traditional paper–pencil tests. This supports the assumption that the main advantage of the presented experimental problems is that it allows students to integrate practical experimental activities during their first semester. The learning process is obviously improved by our more comprehensive experimental exercises with smartphones.
For university teachers who would like to use the new exercise format in their courses, they will have to get their students used to successfully working with smartphones on homework assignments with patience and well-formulated exercises and tasks. The advantages are that the new homework problem type makes learning more varied and that students develop their competencies by checking physical models with their own experiments.
6. Compliance with ethical standards
All procedures performed in studies involving human participants were in accordance with the ethical standards of the institutional and/or national research committee and with the 1964 Helsinki declaration and its later amendments or comparable ethical standards. We are in accordance with the principles outlined in the ethical policy of the journal Physics Education. The study was approved by the study committee of the Faculty for Physics and Earth Sciences at Leipzig University on 30 January 2018.
Acknowledgments
We would like to thank Professor Dr C S Schnohr and PD Dr H von Wenckstern (both Felix-Bloch-Institute for Solid State Physics, University of Leipzig) for their support of this study, as well as all students of the mechanics courses at our faculty during the winter terms 2020/2021 and 2021/22 for their active participation in the study and the positive feedback on the experimental exercises with the smart physics lab. Andreas Kaps is grateful to the University of Leipzig for financially supporting the fellowship of the doctorate.
Data availability statement
The data generated and/or analysed during the current study are not publicly available for legal/ethical reasons but are available from the corresponding author on reasonable request.
Biographies
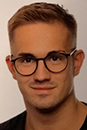
Andreas Kaps PhD student, is a search associate in the Didactics of Physics Group at the University of Leipzig. He has a fellowship from the University Leipzig for his doctorate. His research focuses on smartphone-based experimental exercises to foster the conceptual understanding of the students.
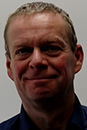
Frank Stallmach, PhD with postdoctoral lecturing qualification, is a lecturer in experimental physics in the Didactics of Physics group at the University of Leipzig. He leads projects on the development of digital teaching and learning environments for university physics education. His current research interests include the use of smartphones as experimental tools in physics education and the establishment of active engegament teaching and learning formats in basic physics lectures.