Abstract
In this paper we describe the STEAM pedagogy developed under the European Union's Horizon 2020 project iMuSciCA (interactive music science collaborative activities). We present the pedagogical model this interdisciplinary pedagogy is based on and we give a concrete example of lesson scenario in which this pedagogy is applied. In particular we show how this interdisciplinary pedagogy can strengthen the understanding of physics concepts and processes by connecting them to corresponding concepts and procedures typical of other disciplines like engineering and music.
Export citation and abstract BibTeX RIS
1. Introduction
The European project iMuSciCA developed a STEAM pedagogy in which physics, engineering, mathematics and music strengthen each other. Through an ICT workbench (https://workbench.imuscica.eu/) and lesson scenarios students are stimulated to go through paths in which they discover connections between concepts and ways of working typical for these different disciplines. A set of iMuSciCA scenarios can be found at https://lms.imuscica.eu/moodle. These have been piloted in Belgian, French and Greece secondary schools mostly during physics and STEM lessons, depending on the national curriculum. Teachers and pupils generally received iMuSciCA with enthusiasm. Data collected during piloting about the usability and effectiveness of the iMuSciCA workbench and scenarios are currently being analyzed and will be published later (more information about the project can be found at www.imuscica.eu).
The iMuSciCA pedagogy applies a model similar to the one reported in [1]: this model is based on two dimensions in which concepts and process skills from the various disciplines are (i) horizontally connected and (ii) conceptually anchored in a vertical structuring phase. These two dimensions are actually intertwined within the iMuSciCA scenarios, as one can start from specific questions or tasks typical of a specific discipline (vertical anchoring), connect to another discipline (horizontal connection), formulate then a new specific question or task typical of that discipline (again vertical anchoring) and so on (figure 1).
Figure 1. The interdisciplinary STEAM pedagogy of iMuSciCA showing the horizontal connections between disciplines and the vertical anchoring.
Download figure:
Standard image High-resolution imageIn this way the iMuSciCA pedagogy helps make the concepts of each specific discipline explicit to students. We choose therefore for an interdisciplinary approach according to [2] in which connections are made between the subjects, but where the subjects remain identifiable. In iMuSciCA we start from concepts of a certain discipline (e.g. physics, music, engineering, maths) and we move towards connected concepts in another discipline, thus showing the relevance of the existing interrelations between them.
The iMuSciCA pedagogy and scenarios are in fact a reflection of the way physics, engineering, math and music influence each other in the real world:
- it is often the case that physics concepts are applied by engineers to develop new technologies, or that engineering is used to build experimental physics material;
- similarly, mathematics is used in physics and engineering to model ideas and concepts; while research in mathematics further develops also thanks to the influence of scientific disciplines;
- on the other hand, although at first sight music appears to have another nature compared to scientific disciplines, there are many connections with physics, engineering and maths: just think about the technologies needed to build a sound synthesizer, or about the scientific explanation of sound waves and eigenfrequencies, etc.
Bringing these 'real' connections into the classroom has the potential to contribute to the development of the so-called 21st century skills like collaboration and creativity, problem solving, etc. which, in their turn, can increase the achievements in specific subjects [3]. In the specific case of STEAM it is the art which introduces a complementary approach to creativity, compared to that of science and engineering.
In this article we focus on the way iMuSciCA strengthens the understanding of concepts and processes typical of physics by connecting it to other disciplines, in the case of engineering and music.
2. Example scenario 'Design & play a guitar in your own tuning'
2.1. Scenario overview
We describe the lesson scenario 'Design & play a guitar in your own tuning—that will work even if you cannot play a guitar' as an example of the iMuSciCA STEAM pedagogy.
This scenario is built around the concept map shown in figure 2 and it is aimed at students in the age-range 14–18 years old. From the point of view of physics tuning strings is a question of varying the value of the influence factors which determine the frequency of a string. In order to strengthen the understanding of these physics concepts we applied the iMuSciCA STEAM pedagogy by connecting the physics concepts to related insights in the engineering and musical fields. In order to make the student experience these connections one can think of different learning paths, starting from one discipline and connecting to the others. Whatever path one will follow, it will start in a certain discipline, turn at some point to another one and come back over and over again, until one understands the problem more deeply, thanks to the different viewpoints, and can solve it.
Figure 2. The concept map of scenario Design & play a guitar in your own tuning.
Download figure:
Standard image High-resolution imageWe present here one possible path the students can go through when working on this STEAM task. The students will go in this example from musical questions towards engineering and scientific ones. The goal of this scenario is indeed to play a guitar, which is clearly a music-related activity. However, the idea is to make this task feasible for all students, even those who might know nothing about playing music. Therefore we propose a learning path which brings the student to engineering: i.e. design a guitar with a special tuning, so that it will be possible to play it without holding knowledge about the guitar chords. However it becomes soon evident that some scientific understanding of the underlying physics is necessary in order to proceed in this task. In order to understand what they have to do, students have to investigate the physical parameters of strings (like thickness, length, tension, nature of the material...) and how these influence the pitch of the produced tone.
2.1.1. The 3D virtual instrument design on the iMuSciCA workbench.
iMuSciCA provides students an online ICT-workbench where they can find virtual tools and activity environments to experiment with in the mentioned STEAM fields. One specific activity environment will be crucial for the presented task: the 3D Virtual Instrument Design. When students open it they get a standard tuned acoustic guitar, but they can alter the following parameters: the nature of the material the string is made off, the density, the thickness and tension of the string (figure 3). Therefore they can experiment by tuning the guitar in another way. In order to analyze the influence of the string parameters on the frequency produced, students will use the virtual monochord on the same environment, as the length of the strings can be changed there as well, while it is fixed in the case of the guitar.
Figure 3. The 3D virtual instrument design tool with the guitar on the iMuSciCA ICT-workbench (workbench.imuscica.eu).
Download figure:
Standard image High-resolution imageIn the following we will illustrate the proposed example learning path in more details. We will also underline the connections between concepts in the different disciplines: physics, engineering and music.
2.2. Scenario of a possible learning path
Step 1—Music: Perform with a guitar that is tuned in a way that it can easily be played.
The idea is that the performance should be feasible even for a student which is not a guitar player. So the task does not consist in designing a standard classical guitar, but one that is tuned in a way that it makes it easy to be played. This could be done, for example, by tuning the guitar so that it can be played just by striking simultaneously all the strings together on their full length or by altering the length of the bunch of strings all together (thus not needing to form chords with the fingers like real guitar players do). You can achieve this when you tune the different strings in a way that the strings produce sounding intervals like an octave or a fifth (which will sound harmonic). A lot of tuning systems used in the folk music are based on this idea (like e.g. the DADAAD, a variation of the open D tuning) and students can look them up as a good starting point to tune the guitar they have in mind.
Step 1, which is clearly music related, is both the starting point and the goal of this STEAM-task. Students will work with the virtual guitar on the 3D Virtual Instrument Design of the iMuSciCA workbench. However the use in parallel of a real guitar is also advised. Indeed, while a virtual guitar can be tuned in a way that cannot be done with a real one, using both virtual and real experiments enhances student's conceptual understanding [4].
This first step brings us quite immediately to the next one: as such a guitar does not exist we will first need to design one. However the structure of the learning path will bring us back to this music step later on.
Step 2—Engineering: Design a guitar that is tuned in a way that it can easily be played.
This is clearly an engineering task as it is about planning, modelling and concretely realizing such a guitar. The goal is to simulate such a virtual guitar using an existing model which is actually based on scientific principles. At this stage it is not necessary to have a deep knowledge of those principles: being able to handle with a series of parameters influencing each other is enough. One option could be just to make students aware that there are correlations between pitch and certain parameters like length, tension, linear mass density of a string (without however knowing the precise relations). The teacher could give the students in that case an excel file as a 'black box' in which the correct relations are embedded: the students just insert the desired values of frequency and typical values of linear mass density and length (of the guitar strings) and they get the needed value of the string tension back. They can then use these values to design their guitar on the 3D Virtual Instrument Design of the iMuSciCA workbench.
However, if we want the students to deeply understand the relation between pitch and the above parameters, we need to go further to the next step, which is related to the physics of tone.
Step 3—Physics: Which influence factors determine the pitch of the string?
In this step students inquire the precise physics relations between the parameters which are used in step 2 and the frequency produced on a string. The students can verify or even 'discover' Mersenne's law [5, 6]:

with: f = frequency (Hz); L = length (m); F = tension (N); µ = linear mass density (kg/m).
The inquiry can be performed at two levels: first qualitatively and then quantitatively. Four influence factors can be inquired:
- 1.influence of length on the frequency
- 2.influence of tension on the frequency
- 3.influence of thickness on the frequency
- 4.influence of material type (and mass density) on the frequency.
The last two inquiries are chosen instead of the linear mass density, which is actually included in Mersenne's law, as those two parameters (thickness and material) are easier to be controlled. In doing this we take into account that:
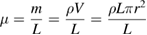
with m = mass (kg); V = volume (m3); 𝜌 = mass density (kg/m3); r = radius (m). In section 2.3 we explain this inquiry in more detail.
Step 4—Engineering: apply these insights on the influence factors to virtually tune a guitar.
At this point students can go back to the engineering field in order to apply the acquired knowledge to concretely design their virtual guitar. As announced in step 2, they could use e.g. an excel file embedding the physics relations of step 3, insert the values of the desired frequencies for the strings of their special guitar and the typical values of linear mass density (or radius and material or mass density) and length and get the needed value of the string tension. Students could experiment by inserting values of linear mass density and length on the virtual guitar which differ from typical values used for real guitars or which are simply not available for real strings. This might also lead to values of the tension which might not be feasible with real strings. That's the interesting side of using a virtual instrument, i.e. exploring the 'extremes' of certain parameters, what cannot be done with a real guitar (e.g. if one increases the tension of a string there's a certain limit upon which the string will break).
Step 5—Music: perform by playing the tuned guitar.
Finally students can go back to the field of music they came from and use the designed and tuned guitar to perform by playing it.
2.3. Detailed description of the activities included in step 3—physics
For this part of the activity students use the virtual monochord on the iMuSciCA 3D Virtual Instrument Design, which allows better control of all parameters compared to the guitar. First students are asked to qualitatively investigate the influence of length, tension, thickness and material type on the frequency: they can just freely try to alter these parameters one at a time and measure how the frequency of the fundamental harmonic changes using e.g. the 2D Sound Visualisation (figure 4). At this point students can start the quantitative analysis.
Figure 4. The 3D virtual instrument design tool with the monochord (left) and the 2D sound visualisation (right) on the iMuSciCA ICT-workbench (workbench.imuscica.eu).
Download figure:
Standard image High-resolution image2.3.1. Length.
Students will first measure the frequency of the fundamental f 1 produced by a certain starting length L of the string and then change it to e.g. L/2, L/3, etc. They measure the corresponding frequencies f n and calculate the ratio f n/f 1 and the product Ln · f n. Based on the results they should be able to identify the relation between length and frequency f ~ 1/L.
2.3.2. Thickness.
Students will follow a similar procedure as above, but this time they will change the starting radius rn into e.g. 2r1, 3r1. Again they will measure the frequency and calculate f n/f 1 and rn · f n, based on which they should be able to find out the relation f ~ 1/r.
2.3.3. Tension and mass density.
Similarly students will start changing the tension and separately the density respectively from a value F1 to 2F1, 3F1, etc and from ρ1 to 2ρ1, 3ρ1 in order to recognize the relations:

and

this time they will have to focus on the frequencies f n measured for tension values like 4F1, 9F1 etc and for mass densities like 4ρ1, 9ρ1 etc, as such values easily allow us to identify a square root dependence.
3. How the iMuSciCA STEAM pedagogy strengthens the understanding of physics
By connecting physics concepts to engineering and music ones, the iMuSciCA STEAM pedagogy can help making physics more relevant to students and to deepen their understanding of it. The proposed STEAM pedagogy does not integrate the different fields in a way they are not distinguishable anymore, on the contrary: it makes the nature of those fields more explicit to students and it connects them through a relevant and wider context, which reflects the real STEAM world. This has the potential to benefit student's understanding of and motivation for physics as they see how physics works, what it does and how it is related to other disciplines in real contexts. In order to support this process it is important to stimulate reflection by students after completing a certain activity: a reflection phase, in which students tell to each other and to the teacher what they have learned, is a good example of vertical anchoring in a certain discipline.
4. Conclusion
We presented a STEAM pedagogical model based on interdisciplinarity rather than integration. This model makes it possible to connect concepts and procedures which are typical of different disciplines, without losing in depth and cohesion inside each discipline. As reported in [7–9] integration and interdisciplinarity are not at all synonym of each other. On the contrary, by following an integrated approach one risks to miss the understanding of the nature of each separate discipline, while an interdisciplinary approach helps precisely recognizing the concepts and processes which are typical of different fields. Interdisciplinary STEAM education is far closer to the real STEAM world outside school, than integrated STEAM is: indeed in the real world of research and industry different people with different backgrounds are working together to solve problems. These people need not to know everything, but rather must be able to talk to each other, by bridging the gap between disciplines. The iMuSciCA STEAM education pedagogy wants precisely to realize this connection inside school, thus reflecting what's happening in the real STEAM world. In this sense this pedagogy makes the relevant connections between STEAM disciplines explicit to students while showing respect for the peculiarity of each separate field like reported in [10].
Acknowledgments
The iMuSciCA project has received funding from the European Union's Horizon 2020 research and innovation programme under grant agreement No 731861. The authors would like to thank their colleague Jeroen Op den Kelder for his technical support and especially for the realization of the figures included in this paper. Special thanks also to colleague Katrien Vyvey for her contribution in helping developing the physics related part of the scenario 'Design & play a guitar in your own tuning'.
Biographies

Dr. Erica Andreotti (female) is researcher in the group 'Art of Teaching' (former Centre for Subject Matter Teaching) of the University Colleges Leuven-Limburg, Belgium. Art of teaching is a research unit focused on the development of Pedagogical Content Knowledge (PCK). Erica is involved in research and professional development on science and STE(A)M education. She has coordinated national and international projects like Quantum SpinOff (EU LLP) and the Flemish STEM Network for secondary schools (funded by the Flemish Ministry of Education). Erica is and has been also involved in other European and national STE(A)M education projects among which the H2020 iMuSciCA. Erica previously worked as doctoral and postdoctoral researcher in Italy, holds a Ph.D. in nuclear and particle physics and carried out (post)doctoral research in the framework of international fundamental research experiments (located at the Gran Sasso National Laboratory, Italy) and for the EU research institute JRC-IRMM (Institute for Reference Materials and Measurements of the Joint Research Centre).

Prof. Renaat Frans (male) lectures Physics Education at the Teacher Education Department of the University Colleges Leuven-Limburg, Belgium. He founded the Expertise Centre Art of Teaching (former Centre for Subject Matter Teaching), a research unit focused on the development of Pedagogical Content Knowledge (PCK). In the last decennium the group grew gradually and currently researchers work at several national and international research projects. The group is specialized in pedagogical questions concerning interdisciplinary teaching within the different sciences and where the sciences meet technology and arts. To disseminate the findings of the centre, Renaat Frans participates with his team in many national and international conferences and organizes courses and workshops for in-service teachers, where hundreds of teachers each year are trained into new pedagogical insights related to STEM and STEAM education. Together with Erica Andreotti, he coordinated the Flemish STEM Network for secondary schools, which was in fact part of the Flemish educational policy to implement STEM in the Flemish Education system. On a policy level, Renaat Frans is consulted for curriculum development and advices for educational policies related to STEM and STEAM.