Abstract
In this paper, the Er3+ and Yb3+ co-doped ZnO films deposited by a novel thermal decomposition method under different annealing temperature process have been reported. The effects of annealing temperature on the morphology and properties of the films are systematically studied. The resulting spectra demonstrate that the Er3+ and Yb3+ co-doped ZnO films possessed the property of up-conversion, converting IR light into visible light that can be absorbed by amorphous silicon solar cell. After all, inner photoelectric effect of the Er3+ and Yb3+ co-doped ZnO films in the amorphous as a light scattering layer are also found with an infrared 980 nm laser as excitation source.
Export citation and abstract BibTeX RIS
1. Introduction
In recent years, research on transparent metallic oxides with a wide energy gap has developed tremendously because of their wide applications in the field of spintronics, information storage, optoelectronics, and photovoltaic devices.1–5) Among the promising large band gap semiconductors based on metallic oxides, ZnO is considered to be an excellent photonic material for amorphous silicon solar cell due to fulfill semiconducting and optical properties, with transparency in the visible range6) and potential impact in the light conversion into electric power. It is also well known that the ZnO thin film can intensify the light absorption and has effective light trapping action, which is favorable to improve the amorphous silicon solar cell.7,8) However, light-absorbing materials developed for solar cells cannot efficiently absorb NIR light. So it is necessary to explore how to synthesis the materials absorbed NIR light.
In order to achieve the harvesting of the NIR part of sunlight, wide band gap materials doped with trivalent rare earth (RE) ions have been currently under development. The idea is to convert the NIR light to high energy photons that can be absorbed by the solar cell.9) For example, TiO2:Er3+,Yb3+ nanocomposite layer have been applied to dye sensitized solar cell to improve the efficiency of the dye-sensitized solar cell (DSSC).10,11) Theoretical estimation shows about 5% increase of the energy conversion efficiency by adding an upconversion wide band gap semiconducting oxide doped with RE ions on the top of a silicon-based conventional solar cell.12) This perspective has led to an intense research activity on upconversion fluorescent materials, which are substances that emit visible light by irradiating NIR as an exciting light. Activators, such as Er3+,13) Ho3+,14) and Tm3+,15) function as the upconversion fluorescent materials.16) Yb3+ ion is usually co-doped as a sensitizer because it has energy bands at 980 nm (2F5/2 → 2F7/2).17–20) In particular, transparent metallic oxides co-doped with Yb3+ and Er3+,21) can efficiently absorb NIR light, such as 980-nm laser light, after which they exhibit up-converted luminescence in the visible range. Here we report the Er3+ and Yb3+ co-doped ZnO films (EYZO films) with different annealing temperatures prepared by an improved ultrasonic spray pyrolysis equipment. The EYZO thin films are deposited onto the silica glass substrates, and the morphology, structure of the films are characterized by atomic force microscopy (AFM), X-ray diffraction (XRD). The effects of annealing temperatures on optical properties are observed and discussed. Simultaneously, we improve the photoelectric performance of amorphous silicon solar cell by introducing an upconversion layer. Under AM1.0 G filtered illumination for a calibrated solar simulator with an overall intensity of 100 mA·cm−2, the conversion efficiency of the amorphous silicon solar cell containing the Er3+ and Yb3+ co-doped ZnO films reach to the optimal values: 4.27%, about 3.6% increasing with pure ZnO structure.
2. Experimental procedure
2.1. Materials
Yb2O3 (99.99%), Er2O3 (99.99%), zinc acetate [Zn(CH3COO)22H2O, analytical reagent (AR)], ethanol (AR), hydrochloric acid (HCL,GR,40%), are supplied by the Sinopharm Chemical Reagent. All the chemicals are used as received without any further purification. Deionized water is used in all experiments.
2.2. Fabrication of the Er3+ and Yb3+ co-doped ZnO films
The schematic diagram of the ultrasonic spray pyrolysis equipment (CQUTUSP-II) is shown in Fig. 1. The starting solution is atomized into fine and uniform droplets using an ultrasonic atomizer, the atomized particles transport to the space near the silica glass substrate carried by the carrier gas.22–24) Then the moisture of droplets starts to evaporate under the action of high temperature. After that, the gaseous contacts the substrate. Finally, the film grows on the substrate. The substrate is placed onto a rotatable stage which ensures the uniform growth of the thin film. An upside down mounted substrate and a vertical feeding of the solution is used in order to make use of gravity to screen out the large droplets leaving the nozzle and to concentrate the same size of droplets on the substrate.
Fig. 1. Schematic diagram of the CQUT USP-II system.
Download figure:
Standard image High-resolution imageTo prepare the Er3+ and Yb3+ co-doped ZnO films, Zn(CH3COO)22H2O, and ErCl3, YbCl3 were mixed in de-ionized water to make a precursor solution. Er3+ doping concentrations were set at 2% in molarities, while Yb3+ doping concentrations were set at 6%. An appropriate amount of acetic acid was added into the solution to avoid the formation of milky precipitate of hydroxides. Before the experiment, the silica glass substrates, were cleaned successively with hydrochloric acid, ethanol and distilled water in an ultrasonic cleaning system. The distance of source substrate was fixed at 2 cm and the substrates temperatures were fixed at 400 °C. As the precursor droplets arrive on the heated substrate, a pyrolytic decomposition process occurs and high quality EYZO films were produced. The chemical reactions followed during the pyrolytic decomposition process25) are described as follows:
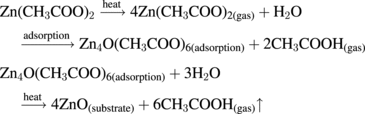
2.3. Characterizations
Structures of the samples are investigated by XRD using a X'TRA (ARL) equipment provided with Cu tube with Kα radiation at 1.54056 Å in the range of . Atomic force microscopy (Veeco Multimode III) is used to analyze the surface morphology of the samples. The AFM images are recorded over a scan area of 10 × 10 µm2 of the film surface. Luminescence spectra are obtained by the Omin-λ3007 Spectrophotometer with a photomultiplier tube equipped with a 980 nm laser diode as the excitation source. The photocurrent of the samples are measured by CHI660E when the device is illuminated by a 980 nm laser. All measurements are performed at room temperature.
3. Results and discussion
3.1. Phases and morphologies of the prepared samples
To investigate the crystalline quality of the EYZO thin films with various annealing temperatures, XRD analysis is carried out and the results are shown in Fig. 2. The XRD patterns of the films indicate the existence of a ZnO single phase with a hexagonal wurtzite structure (JCPDS No. 36-1451). The strong and dominating nature of the peak corresponding to the (002) reflection indicates the preferential c-axis orientation of crystallites which are reported to have the lowest surface energy.26) Moreover, the intensity of the peaks gradually increases with the increase of annealing temperatures, which indicate that the increase in annealing temperature enhances the crystallinity of the films. No impurity peaks can be identified from the XRD pattern, indicating that the sample has been synthesized into pure hexagonal ZnO.
Fig. 2. XRD patterns of the films prepared at various annealing temperatures.
Download figure:
Standard image High-resolution imageThe surface morphology of the films was characterized by AFM, Fig. 3 shows AFM images of EYZO thin films prepared with different annealing temperatures and deposited at 400 °C for 45 min. The images are recorded on 10 × 10 µm2 planar in contact mode. The surface morphology of the film shows uniform and continuous grains without any voids on the surface. Figure 3(d) illustrates the root-mean-square (RMS) surface roughness decreases from 143.67 to 68.22 nm in the investigated thickness range. Close monitoring of the AFM images of films with different annealing temperature further reveals that crystalline quality of films upgrades slightly with increasing the annealing temperature.
Fig. 3. AFM 2D and 3D images of the Er3+ and Yb3+ co-doped ZnO films with various annealing temperatures: (a) 600, (b) 700, (c) 800 °C and (d) RMS roughness with various annealing temperatures.
Download figure:
Standard image High-resolution image3.2. Photoluminescence: Energy transfer
Up-conversion photoluminescence excitation was performed through a 980 nm laser. As we can see from Fig. 4, the up-conversion luminescence can be enhanced by increasing the annealing temperature. And when the annealing temperature reached 900 °C, there is no emission peak observed. The observed peaks located at 527, 546, and 660 nm are exactly attributed to the 2H11/2 → 4I15/2, 4S3/2 → 4I15/2, 4F9/2 → 4F15/2 transitions of Er3+ ion. It is well known, the main absorption wavelength of the amorphous silicon solar cell is from 400 to 800 nm, which indicates the EYZO films convert the "waste light" from 980 nm laser to visible light which can be utilized by the amorphous silicon solar cell. Yb3+ ion is usually co-doped as a sensitizer so as to enhance up-conversion luminescence efficiency for its large absorption cross-section in the 900 nm NIR region, correspond to the transition of 2F5/2 → 2F7/2.
Fig. 4. PL spectra of the the Er3+ and Yb3+ co-doped ZnO films prepared at various annealing temperatures.
Download figure:
Standard image High-resolution imageThe energy level of Er3+ and Yb3+ ions as well as the proposed up-conversion mechanism are shown in Fig. 5. It is obvious that, for the single 980 nm excitation corresponding to the 2F7/2 → 2F5/2 transition of Yb3+ ion and successive energy transfers from Yb3+ to Er3+, the green emission is ascribed to 2H11/2 → 4I15/2, 4S3/2 → 4I15/2 transitions of Er3+, and the red emission is ascribed to 4F9/2 → 4I15/2.27) In upconversion emission mechanisms, excited-state absorption and energy-transfer excitation have been reported.28–30) Green emission can be explained as follows: a 980 nm laser excites from the ground state 4I15/2 to the excited state 4I11/2. In the excited-state absorption, the photon energy of a 980 nm laser excites from the 4I11/2 level to the 4F9/2 in the Er3+ level. In the energy-transfer excitation, the photon energy transfers from Yb3+(2F5/2 → 2F7/2) to the Er3+(4I11/2 → 4F7/2). Subsequently, a nonradioactive relaxation occurs from the 4F7/2 level to the 2H11/2 level or the 4S3/2 level. Finally, the green emission is observed in 2H11/2 → 4I15/2, 4S3/2 → 4I15/2 transition. Conversely, red emission can be explained as follows: a 980 nm laser excites Er3+ from the ground state 4I15/2 to the excited state 4I11/2. Subsequently, a nonradioactive relaxation occurs from the 4I11/2 level to the 4I13/2. In the excited-state absorption, the photon energy of a 980 nm laser excites from the 4I13/2 level to the 4F9/2 level. In the energy-transfer excitation, the photon energy transfers from Yb3+(2F5/2 → 2F7/2) to the Er3+(4I13/2 → 4F9/2). Finally, the red emission is observed from the 4F9/2 → 4I15/2 transition in the Er3+.
Fig. 5. Mechanisms of Er3+,Yb3+ up-conversion emissions under 980 nm excitation.
Download figure:
Standard image High-resolution image3.3. Photoelectric effect
To study the efficiency of up-conversion for this material, we measure the photovoltaic parameters of the amorphous silicon solar cell containing the Er3+ and Yb3+ co-doped ZnO films. According to photovoltaic properties of the materials shown in Table I and Fig. 6, the ZnO film annealed at 800 °C yielded the best performance among these films with an overall efficiency (η) of 4.27% under the simulated solar irradiation of 100 mA·cm−2. The open-circuit voltage (Voc) and the short-circuit current density (Isc) of sample d is 2.9 V and 2.51 mA·cm−2. Compared with the conversion efficiency (4.12%) of amorphous silicon with pure ZnO structure, the increased conversion efficiency (η, ∼3.6% increase) in the amorphous silicon solar cell containing the EYZO film indicates that this material is better than La–ZnO, Ce–ZnO, Nd–ZnO, and Sm–ZnO materials.31) The improvement in the conversion efficiency is closed to the theoretical estimation (5%) of the reference.12)
Table I. Photoelectric properties of the amorphous silicon solar cell with (a) pure ZnO structure and at different annealing temperatures: (b) 600, (c) 700, and (d) 800 °C.
Sample | Jsc (mA·cm−2) | Voc (V) | FF | η (%) |
---|---|---|---|---|
a | 2.34 | 2.89 | 0.62 | 4.12 |
b | 2.46 | 2.89 | 0.62 | 4.18 |
c | 2.47 | 2.9 | 0.62 | 4.19 |
d | 2.51 | 2.9 | 0.62 | 4.27 |
Fig. 6. Photoelectric properties of the amorphous silicon solar cell with (a) pure ZnO structure and at different annealing temperatures: (b) 600, (c) 700, and (d) 800 °C.
Download figure:
Standard image High-resolution imageIn order to demonstrate unequivocally that introducing the EYZO film on the solar cell leads to photocurrent generation via the upconversion of NIR light, we put the films on the solar cell as showed in Fig. 7. We use 980 nm infrared laser as the excitation source because the amorphous silicon solar cell cannot absorb at the wavelength.32) When the laser turning off, the photocurrent decreases quickly while the photocurrent increase significantly when the laser turning on. No photoelectric effect is observed without excitation from the 980 nm laser. Thus, the generated photocurrent can be solely contributed to the NIR light harvesting via the EYZO film modified the solar cell. The photocurrent is found to increase with the increase of annealing temperature from 600 to 800 °C. Figure 8 shows that transient current of the cells containing the EYZO films increase with increasing excitation power of the 980 nm laser from 53.6 to 180.0 mW. The photocurrent origion of the amorphous silicon solar cell containing the EYZO films under 980 nm excitation can be explained as follows: The Yb3+ ions absorbed the energy from 980 nm laser and the energy transferred from Yb3+ to Er3+, then generated the red light, finally the red light absorbed by the amorphous silicon solar cell. It should be noted that although the up-converting luminescence is emitted in all spatial directions, most of the visible photons are absorbed by the amorphous silicon solar cell because of their strong absorption in the visible-light region.
Fig. 7. Schematic illustration of the amorphous silicon solar cell containing the Er3+ and Yb3+ co-doped ZnO films.
Download figure:
Standard image High-resolution imageFig. 8. Transient photocurrent responses of the amorphous silicon solar cell containing the Er3+ and Yb3+ co-doped ZnO films prepared at various annealing temperatures in dependence of different excitation powers of the 980 nm laser. The inset shows transient photocurrent responses of the amorphous silicon solar cell containing the EYZO films with a 980 nm laser (P = 121.3 mW) as excitation sources.
Download figure:
Standard image High-resolution image4. Conclusions
Er and Yb co-doped thin ZnO films were deposited through an ultrasonic spray pyrolysis equipment, and annealed at different temperatures from 600 to 800 °C to optically activate Er3+ ions. The morphology of the films can be modified by tuning the annealing temperature. Photoluminescence is recorded at room temperature for all films. The annealing treatment appeared to be essential to convert Er3+ ions in optically active centers, and the intensity of the PL mission peaks is the strongest when annealing temperature reached 800 °C. The present results demonstrate the improvement in the near-infrared amorphous silicon solar cell response by introducing ZnO: Er, Yb layer. And the EYZO films provide an effective way to improve the sunlight conversion efficiency for the amorphous silicon solar cells.
Acknowledgments
This work was supported by National Natural Science Foundation of China (11404171), Natural Science Youth Foundation of Jiangsu Province (BK20130865), the Six Categories of Summit Talents of Jiangsu Province of China (2014-XCL-021), and the Natural Science Foundation of the Jiangsu Higher Education Institutions of China (14KJB430020).