Abstract
In this study a novel magnetic composite used as a photocatalyst with combination of zinc oxide nanoparticles and chitosan (ZnO/Fe3O4/CS) was synthesized by a simple co-precipitation method. The role of the prepared magnetic nanocomposite is to improve the removal efficiency of textile dye due to the photocatalytic activity of zinc oxide nanoparticles and reusable capacity of Fe3O4 magnetic nanoparticles. Constituents and structure properties of ZnO/Fe3O4/CS were investigated by scanning electron microscopy (SEM), x-ray diffraction (XRD), Fourier transform infrared spectroscopy (FTIR) and thermogravimetric analysis (TGA). Magnetic property of the prepared composite was determined by vibrating sample magnetometer (VSM). The results demonstrated that ZnO/Fe3O4/CS nanocomposite dramatically improved the removal efficiency of reactive blue 198 dye (RB198) with high photocatalytic activity and easy separation by a permanent magnet. In addition, the photocatalytic activity of the prepared composite was also performed under different parameters such as contact time, initial pH, the amount of composite and initial concentration of RB198. Interestingly, ZnO/Fe3O4/CS nanocomposite still showed high removal efficiency after recycling three times and performed in a real textile dyeing wastewater.
Export citation and abstract BibTeX RIS

Original content from this work may be used under the terms of the Creative Commons Attribution 3.0 licence. Any further distribution of this work must maintain attribution to the author(s) and the title of the work, journal citation and DOI.
1. Introduction
In recent years, global climate change has become a serious issue predominantly caused by environmental pollution from industrial activities, especially in developing countries. Among fields of industries, textile dyeing industries have distributed a huge volume of colored, toxic and non-biodegradable dyestuffs, which is one of the most polluting compounds of clean water [1]. At present, the textile dyeing industry uses thousands of dyes for textile fabrication processes [2]. In addition, 60–70% of used dyestuffs are azo substances, which yields cancer-causing and aquatic animal-poisoning compounds [3]. Reactive blue 198 (RB198) has been known as one of the most widely used in textile manufacture owing to its effective applications [4, 5]. However, RB198 is easily hydrolyzed in water to produce free colored dyes after dyeing process so that there still remains a large amount of RB198 in wastewater discharging to environmental surroundings. This dye is hard to degrade and especially stable to light and heat. Therefore, finding effective methods for treating wastewater containing RB198-like compounds is an important solution to control the environmental pollution.
The adsorption method has been demonstrated as one of the most effective ways for removal of dyestuffs by using non-toxic and biodegradable materials including hydroxyapatite [6], nanoclay, derivations of cellulose [7], bentonite [8] due to high adsorption efficiency, low cost and environmentally-friendly ability. However, the adsorption method just performed their effective adsorption capacity towards some specific types of dyes so that this method still has some drawbacks. Furthermore, semiconductor photocatalysts [9] also have been known as a newly developed advanced oxidation processes which can oxidize a broad range of various dyes because of hydroxyl radicals generation [10]. Zinc oxide, a photocatalyst, is appropriate alternative to TiO2 for the degradation of dyes as its degradation mechanism is similar to that of TiO2 [1, 11–14]. Zinc oxide (ZnO) can oxidize photocatalytically aromatic hydrocarbons of phosphorus, sulphur, nitrogen and halogen to release final non-toxic or easy-to-treat products. Moreover, nanoscale of zinc oxide attracts more attention than that of macroscale zinc oxide due to its special physical and chemical characterizations such as magnetism and electrical conductivity.
In order to improve the degradation efficiency of zinc oxide nanoparticles, a simple effective method is applied to combine a magnetic property of Fe3O4 with zinc oxide nanoparticles using chitosan (CS) as a substrate to create a magnetic composite that could be recycled for several times of usage by an external magnet. Fe3O4 nanoparticle [15, 16], the most popular magnetic material, is widely used for biomedical applications such as drug delivery [17, 18], imaging [19], gene transfection [20] and so on. The core–shell nanocomposite of Mn-doped ZnO and Fe3O4 for photodegradation of textile dye was reported in reference [21]. This nanocomposite exhibited a high de-colorization of reactive blue 198 at neutral pH, however, the photocatalytic activity decreased with increasing and decreasing pH. The low photocatalytic activity at pH smaller than 6 may be due to the decomposition of photoactivity shell of nanocomposite, while the decrease of photocatalytic activity at increasing pH above 7 may be due to the presence of the point of vanishing ZnO charge. Therefore, to improve the photocatalytic activity of ZnO nanoparticle in the wide range of pH, the chitosan was used to coat the ZnO nanoparticle and Fe3O4 nanoparticle.
Chitosan has been known as natural bio-polymeric materials derived from shrimp and crab shells with characterized properties consisting of non-toxic, biodegradation. Besides, chitosan is commonly used as a substrate or carrier in applications of composite for environmental treatment and drug delivery [22]. In this study, a novel synthesized magnetic composite of chitosan and zinc oxide (ZnO/Fe3O4/CS) were prepared and paved the way for environmental applications of reusable photocatalytic materials compared with single photocatalyst of zinc oxide nanoparticles. Additionally, the ZnO/Fe3O4/CS can be recycled using an external magnet to improve applications for the removal of organic textile dyes and real dyeing wastewater.
2. Materials and method
2.1. Materials
Chitosan (90% de-acetylation) was prepared in our lab. Reactive blue 198 (RB 198) and real textile dyeing wastewater were donated by Thanh Cong textile company. All chemicals were analytical grade and used without further purification and all solutions were prepared with deionized water.
2.2. Synthesis of Fe3O4 magnetic nanoparticles
Fe3O4 nanoparticles were successfully fabricated by a co-precipitate method of Fe2+ and Fe3+ in ammonia solution. In this paper, synthesized Fe3O4 was synthesized by the method presented in previous research with some modification [23]. In brief, 0.01 mol of FeCl3.6H2O and 0.005 mol of FeCl2.4.H2O were dissolved into 50 mL of deoxygenated water. Fe3O4 precipitation was obtained by adding ammonia solution 25% at 80 °C for hours under a stirrer. The pH of solution was maintained at 10. Later, the precipitate was achieved by a filtration paper and washed with distilled water and ethanol for several times. Lastly, the obtained precipitate was dried at 90 °C in an oven.
2.3. Synthesis of zinc oxide nanoparticles
There were many methods to synthesize zinc oxide nanoparticles. In this study, zinc oxide nanoparticles were simply fabricated by a sol-gel method with some adjustment [24]. Briefly, 30 mL of triethanolamine (TEA) was added into a beaker containing 20 mL of distilled water. A stirrer was used to mix solution homogeneously. During stirring, 2 mL ethanol was pipetted into the homogeneous TEA solution. Then, 0.5 M zinc acetate solution (2.7 g of Zn(CH3COO)2 into 50 mL H2O) was transferred into TEA solution. The mixture was continuously stirred for 20 min at temperature of 120 °C. Afterwards, 6 mL of NH4OH (25%) was slowly dropped into the solution. An aluminum foil was used to cover the container of the reaction, for light prevention. Immediately, there was a formation of milky precipitate of zinc oxide nanoparticles in the solution. The solution was kept to stand for 30 min to obtain the nanoparticles on the bottom of beaker. The precipitate was achieved by filtration and washed for several times by distilled water and ethanol. Finally, the resulting precipitate was dried at 90° C for 4 h.
2.4. Synthesis of magnetic composite based on zinc oxide nanoparticles and chitosan
In this study the magnetic composite based on zinc oxide nanoparticles and chitosan was synthesized by adding ZnO and Fe3O4 into chitosan matrix using glutaraldehyde as a cross-linker [23]. Firstly, 1% chitosan solution was prepared by dissolving 2.5 g chitosan in acetic acid 2.5%. Then, 1 g of ZnO and 1.25 g of Fe3O4 nanoparticles were added in the 1% chitosan solution and stirred continuously by a mechanical stirrer at 60 °C for 3 h to disperse steadily the particles into chitosan solution. Then, 0.5 mL glutaraldehyde solution was slowly pipetted into the mixture to form gel. After completing reaction, the pH of the mixture was modified to 10 using 2 M NaOH solution. The mixture was stirred and heated on a water bath for 3 h at 60 °C. The magnetic composite was formed and obtained by using a filtration paper. Then, the resulting composite was washed with acetone and distilled water and dried at 90 °C.
2.5. Characterization
The x-ray diffraction was carried out in X'Pert Pro MRD (PANanalytical, Netherlands) at room temperature with Cu-Kα radiation (λ = 0.154 nm) and an incident angle (2θ), ramping from 2 to 80° at a rate of 50 s/step. Magnetic measurement of the product was conducted on a vibrating sample magnetometer (VSM-5 Model, MicroSense Co., Ltd). Scanning electron microscopy (SEM) and Fourier transform infrared spectroscopy (FTIR) were used to characterize the morphology and structure of prepared material. Thermogravimetric analysis (TGA) was made by using a Mettler-Toledo thermo-gravimetric analyzer.
2.6. Testing of photocatalytic activity of ZnO/Fe3O4/CS composite
In order to show potential environmental applications of ZnO/Fe3O4/CS composite for photocatalytic degradation of RB198, all experiments were carried out under UV light irradiation. 0.25 g of ZnO/Fe3O4/CS composite was added into a beaker containing 50 mL of RB198 solution with various initial concentrations (10, 20, 30, 40, 50 and 60 mg L−1). Then, the solution was regularly mixed by a stirrer bar under UV light irradiation. After a certain time, the suspension of material was separated by a permanent magnet. The solution was obtained to analyze optical density by using UV–vis spectrophotometer at a wavelength of 625 nm. The degradation efficiency of RB198 was calculated as follows
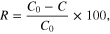
where C0 and C are concentrations of the dye solution before and after photo irradiation.
2.6.1. Effect of illumination time and initial concentration of RB198
The influence of illumination time and initial concentration of RB198 on the ability of photo-degradation of RB198 was studied in different time intervals with various RB198 concentrations. 0.25 g of composite was added into each of 100 mL beakers containing 50 mL of RB198 solution with concentrations of 10, 20, 30, 40, 50 and 60 mg L−1. A stirrer (300 rpm) was used under UV light irradiation system to make sure the composite disperse regularly into the solution. After different times ranging from 5 to 80 min, the resulting solutions were separated by using a permanent magnet. The optical density of solutions was determined by using UV–vis spectrophotometer at wavelength of 625 nm.
2.6.2. Effect of the amount of composite loading
The effect of ZnO/Fe3O4/CS composite dosage on photodegradation efficiency of RB198 was carried out with the same illumination time and initial concentration of RB198. Determined amounts of ZnO/Fe3O4/CS composite (0.1, 0.25, 0.4 and 0.5 g) were transferred into a 100 mL beaker containing 50 mL of 30 mg L−1 RB198 solution. A magnetic stirrer was used under UV light irradiation system in a time interval. After separation, the optical density of solutions was measured.
2.6.3. Effect of initial pH solution
The impact of pH on the photodegradation efficiency of RB19 was examined by adding the composite into the RB198 solution with pH values ranging from 6 to 8. The experiments were performed under a magnetic stirrer and UV light irradiation with the same illumination time (10 min), initial RB198 concentration (30 mg L−1), the amount of the composite (0.25 g). The pH of solution was adjusted by using 0.1 M HCl or 0.1 M NaOH solution. After completing the separation, the optical density of solutions was analyzed.
2.7. Recycling composite
The remainder of the composite after separation from all experiments was gathered in a glass bottle. Then, the composite was washed with distilled water for several times and dried at 90 °C for recycling experiments. 0.25 g of recycled composite was added into 50 mL of RB198 solution with the same optimized parameters such as pH, dosage of composite, initial concentration and illumination. After exposing to UV light, the resulting solutions were separated by a permanent magnet. Finally, the optical density of solutions was determined. The remaining composite was obtained, washed and dried to repeat to recycling experiments. The repetition of recycling was carried out until the photo-degradation efficiency of composite was about 70%.
2.8. Testing catalyst with real textile dyeing wastewater
After investigating the photocatalytic ability of composite with RB198, a performance with real textile dyeing wastewater was also carried out. Real wastewater was used from industrial textile dyeing factory with initial pH of 12. The pH of wastewater was adjusted to the optimized value from following experiments. Then, 0.25 g of composite was transferred to 50 mL of RB198 under UV light irradiation and a magnetic stirrer at 300 rpm. After certain time, the resulting solution was separated and used to measure chemical oxygen demand (COD) in order to determine the total of remaining organic compounds in wastewater.
3. Results and discussion
3.1. Characterization of ZnO/Fe3O4/CS
The scanning electron microscopy (SEM) micrograph of ZnO/Fe3O4/CS was shown in figure 1. As seen, the result of micrograph showed that the surface of the composite was rough with tiny porous structure with opening holes on the surface. Figure 1 also revealed that porous structure of the composite had interconnection between opening holes, which help increase surface area of the catalyst contacting with dye molecules (RB198). In addition, there was one solid phase in the micrograph of the synthesized composite meaning that ZnO and Fe3O4 nanoparticles were relatively well-dispersed into chitosan.
Figure 1. SEM image of prepared ZnO/Fe3O4/CS.
Download figure:
Standard image High-resolution imageThe FTIR graphs of ZnO/Fe3O4/CS and pure chitosan were presented in figure 2. The graphs showed several typical peaks revealing the presence of chitosan as one of the components in structure of ZnO/Fe3O4/CS when compared with pure chitosan [25]. The specific adsorption band at 3423.7 cm−1 was attributed to the stretching vibration of O-H and N-H bonds. The characteristic peak appeared at 1345 cm−1 corresponding to the stretching of C-N of -NHCO, while the peaks at 1055.69 cm−1 and 2923 cm−1 were ascribed to C-OH bond and C-H of alkyl group, respectively. Especially, there was a new typical peak at 1560.87 cm−1 confirming the formation of imine crosslinking as a result of Schiff base reaction between –NH2 of chitosan and −C=O of glutaraldehyde. In addition, the existence of ZnO and Fe3O4 nanoparticles in the composite was demonstrated by the appearance of some new characteristic peaks. The specific adsorption bands at 1413.75 and 645.78 cm−1 were assigned to Zn-O bond. The peaks at 566.77 cm−1 and 419.36 cm−1 were characterized for Fe-O. As result, the FTIR graph proved the contribution of chitosan, ZnO and Fe3O4 nanoparticles, which was correlating to the results of XRD patterns.
Figure 2. FTIR spectra of the photocatalytic composite.
Download figure:
Standard image High-resolution imageThe XRD patterns of ZnO/Fe3O4/CS, Fe3O4, ZnO and chitosan were described in figure 3. As seen, the XRD patterns showed the straight base line and unsharp peaks which indicated that the ZnO/Fe3O4/CS composite was not a well crystalized material compared with separated Fe3O4 and ZnO nanoparticles. This may be due to the existence of chitosan on surface of ZnO and Fe3O4. The typical peaks at 10.12°, 19.7°, 21.5° and 42.2° were assigned to chitosan coating on nanoparticles [26], while the characteristic peaks at 31.7°, 34.5°, 36.2°, 47.5° and 56.7° were ascribed for ZnO in the composite [27]. Moreover, figure 3 also presented that diffractogram of the composite still had peaks revealing the presence of Fe3O4 in structure of the material with specific peaks at 35.5° and 63° [15]. The results of XRD patterns along with FTIR graphs strongly confirmed that the nanocomposite was successfully synthesized with constituents of ZnO, Fe3O4 and chitosan.
Figure 3. XRD patterns of ZnO, Fe3O4 and ZnO/Fe3O4/CS nanoparticles.
Download figure:
Standard image High-resolution imageThe magnetic property of the composite was determined by a vibrating sample magnetometer. Magnetization curves of ZnO/Fe3O4/CS and Fe3O4 were shown in figure 4. Once again, the magnetization curve of ZnO/Fe3O4/CS demonstrated the presence of Fe3O4 in structure of the composite. The curves also revealed the superparamagnetism of Fe3O4 and composite. The saturation magnetization value of Fe3O4 was about 40 emμ g−1. However, the saturation magnetization of ZnO/Fe3O4/CS decreased to about 10 emμ g−1. The decrease of the saturation magnetization of ZnO/Fe3O4/CS may be due to the influence of chitosan after coating on surface of Fe3O4 and the dispersion of Fe3O4 into the matrix of chitosan. Moreover, the results also proved that the saturation magnetization of composite based on contents of Fe3O4 and chitosan.
Figure 4. Magnetization curves of ZnO/ Fe3O4/CS composite and Fe3O4.
Download figure:
Standard image High-resolution imageThe results of thermogravimetric analysis of ZnO/Fe3O4/CS were presented in figure 5. Thermogravimetric (TG) and differential scanning calorimetry (DSC) curves are two characterized curves to determine changes in physical and chemical properties of composite when increasing temperature in air at a heating rate of 10 °C min−1. As seen, weight loss of composite was about 45% when heating from 40–558 °C. The process of weight loss underwent two stages. For the first stage, weight loss of the composite was about 7% meaning the evaporation of water and volatile molecules in synthesized composite with temperature heating from 40–200 °C. Moreover, an endothermic peak in DSC curve revealed that a phase transition from liquid state to vapor state occurred at 55 °C. For the second stages with temperature ranging from 200–558 °C, the weight loss of prepared composite was 38% due to the decomposition of chitosan. There was the presence of two exothermic peaks at 310 °C and 440 °C. Additionally, there was no significant difference in weight loss of the prepared composite. This may be because the melting point of ZnO and Fe3O4 was higher than 558 °C. Therefore, remainder of the composite could be attributed to Fe3O4 and ZnO with weight of 55%.
Figure 5. Thermogravimetric analysis of ZnO/Fe3O4/CS: a) TG and b) DSC curves.
Download figure:
Standard image High-resolution image3.2. Testing photocatalytic activity of ZnO/Fe3O4/CS composite
3.2.1. Effect of illumination time
The influence of illumination time and initial RB198 concentration on decolorization of RB198 was shown in figure 6. The decolorization of RB198 proportionally depended on the illumination time of the UV light system. The decolorization efficiency augmented responsively with the increase of exposing time of ZnO/Fe3O4/CS under UV light irradiation. Generally, the decolorization efficiency reached 100% after a certain illumination time with different concentrations. Specifically, the efficiency of 100% reached at illumination time of 40, 50, 80, 90, 100 and 120 min corresponding to RB198 concentration of 10, 20, 30, 40, 50 and 60 mg L−1, respectively. As it can be seen, the equilibrium time was faster when the RB198 concentrations were higher. In addition, figure 7 showed the influence of illumination time on optical density of RB198 at concentration of 30 mg L−1. When the time increased from 10 to 30 min, the optical density of RB198 augmented corresponsively. Once again, this result indicated the reduction of RB198 concentration leading to the decrease of its optical density.
Figure 6. Decoloration (%) of ZnO/Fe3O4/CS at different time and different initial RB198 concentration (0.25 g ZnO/Fe3O4/CS, solution volume: 50 mL and pH = 6).
Download figure:
Standard image High-resolution imageFigure 7. Time-dependent UV–vis absorption spectra for decolorization of RB198 (0.25 g of ZnO/Fe3O4/CS, solution volume: 50 mL, pH = 6, initial RB198 concentration: 30 mg L−1).
Download figure:
Standard image High-resolution image3.2.2. The effect of initial RB198 concentration
The effect of initial dye concentration was investigated by adjusting the initial concentration from 10 to 60 mg L−1 and using 0.25 mg of catalyst and 50 ml of dye solution under UV light for 10 min. The obtained results were showed in figure 8. It can be seen that the photodegradation efficiency of RB 198 was inversely proportional to its concentration, meaning that the lower of the dye concentration, the higher photodegradation efficiency. Furthermore, the increase of initial dye concentration from 10 to 60 mg L−1 also led to the reduction of photodegradation efficiency of RB198 from 82.3% to 38.9% after 10 min of UV irradiation. The mechanism of photodegradation was explained by the formation of critical species—hydroxyl radical, which was released during degradation process under UV light irradiation. Therefore, the initial concentration at higher concentration had stronger color intensity due to the representation of dye molecules in solution. This led to the obstacles between the active contact surface of ZnO/Fe3O4/CS and RB198 molecules causing the decrease of generation of due to the fewer active sites for the adsorption of
Additionally, the route of photons going into the RB198 solution was shortened under constant intensity of UV light, which caused fewer photons interacting with catalyst surface. This was a result of reducing hydroxyl radicals that can attack RB198; therefore it leads to a decrease of photodegradation efficiency.
Figure 8. Effect of initial dye concentration on the photo-degradation efficiency of RB198 (0.25 g ZnO/Fe3O4/CS, solution volume: 50 mL, pH = 6 and illumination time: 10 min).
Download figure:
Standard image High-resolution image3.2.3. Effect of catalyst loading
The effect of ZnO/Fe3O4/CS dosage on the photodegradation efficiency of RB198 was observed by taking different amounts of catalyst ranging from 0.1 to 0.5 mg into 50 mL of 30 mg L−1 dye solution with pH of distilled water under UV light irradiation for 10 min. The obtained results are depicted in figure 9. As seen, when increasing the catalyst loading from 0.1 to 0.5 mg, the photodegradation efficiency of RB198 also raised from 42% to 100%. This may be explained due to the increase of contact area between active regions with RB198 corresponding to the raise of ZnO/Fe3O4/CS dosage. Particularly, the highest photodegradation efficiency was about 100% at 0.5 gram of the composite, while the lowest photodegradation efficiency was 41.6% at 0.1 g of the composite.
Figure 9. Effect of catalyst loading on the photodegradation efficiency of RB 198 (solution volume: 50 mL, pH = 6 and illumination time: 10 min, initial RB198 concentration: 30 mg L−1).
Download figure:
Standard image High-resolution imageMoreover, figure 10 also presented the UV–vis absorption spectra for degradation of RB 198 by using different ZnO/Fe3O4/CS catalyst dosage. As can be seen, the UV–vis absorption spectrum of RB198 at 0 g was highest due to no active regions to attack RB198. On the other hand, when raising the amount of ZnO/Fe3O4/CS catalyst dosage from 0.1 to 0.5 g, the absorption spectrum of RB198 trended to induce. This was because of the increase the active sites of hydroxyl radicals. Therefore, the lowest absorption spectrum of RB198 was at 0.5 g.
Figure 10. Catalyst loading-dependent UV–vis absorption spectra for decolorization of RB198 (solution volume: 50 mL, pH = 6 and illumination time: 10 min, initial RB198 concentration: 30 mg L−1).
Download figure:
Standard image High-resolution image3.2.4. Effect of pH of RB198 solution
It has been well-known that the pH value could influence the rate of photocatalytic degradation of some organic compounds. The impact of pH of RB198 was investigated in this study. The experiments were carried out in the pH range intervals of 6–8 by using 0.25 g of ZnO/Fe3O4/CS catalyst and 50 mL of 30 mg L−1 RB198 solution under UV light irradiation for 10 min. Figure 11 demonstrated the result of photodegradation efficiency of RB198 with different pH values. The result revealed that the photodegradation efficiency decreases with the increase of pH value. Concretely, the photodegradation efficiency reached to 85% at low pH value (pH = 6). Inversely, the lowest photodegradation efficiency was 57.76% at pH of 8. This indicated that photodegradation efficiency was higher in an acidic medium than that of a basic medium. Because chitosan matrix can be swollen in acidic medium due to the hydrogen bonds between H+ and –OH groups in chitosan. Therefore, the swollen chitosan matrix made the porous holes larger in acidic medium, which increased the contact area with BR198 in solution. Besides, NH2 groups of chitosan can be easily positive charged by protonation of H+ protons in acidic solution that help attract negative charged RB198 molecules owning to electrostatic interaction.
Figure 11. Effect of pH of dye solution on the photodegradation efficiency of RB 198 (solution volume: 50 mL, illumination time: 10 min, initial RB198 concentration: 30 mg L−1 and dosage of ZnO/Fe3O4/C: 0.25 g).
Download figure:
Standard image High-resolution image3.2.5. Recycling experiments of ZnO/Fe3O4/CS catalyst
Favorably, the ZnO/Fe3O4/CS composite has magnetic activity which can easily recycle by using the magnet. Therefore, recycling experiments were repeated in several times. The results were shown in figure 12. As seen, the photocatalytic degradation of RB198 decreased from 96.17% to 76.28% after three times of usage. The results proved that ZnO/Fe3O4/CS catalyst can be used as a promising photo-catalytic composite for removal of dye solution with reusable property. In addition, after three times of usage, the decolorization of RB198 still reached over 70%. This indicated that ZnO/Fe3O4/CS was applicable for wastewater treatment. Interestingly, the chemical oxygen demand (COD) value of BR198 was 112 mg L−1 before the treating with ZnO/Fe3O4/CS. However, it significantly decreased to 40 mg L−1 after using the photocatalytic composite for treatment. Once again, the COD value investigated the power of ZnO/Fe3O4/CS in removal of RB198.
Figure 12. The relationship between usage time and decoloration of RB198 by using ZnO/Fe3O4/CS.
Download figure:
Standard image High-resolution image3.2.6. Real wastewater performance
In the present study, the activity of ZnO/Fe3O4/CS photocatalytic composite was tested on real wastewater from Thanh Cong textile and dying company. The COD value was determined before and after treatment to evaluate photodegradation efficiency of Zn/Fe3O4/CS. The results were shown in table 1. As observed, the COD value decreased from 276 to 126 mg L−1 before and after treatment, respectively. The results confirmed that the prepared catalyst can eliminate toxic organic compounds in real wastewater. These results paved a new way for effective removal of real dye wastewater.
Table 1. COD of textile dyeing wastewater before and after treatment.
Photodegradation time (min) | Volume of wastewater (mL) | COD (mg L−1) | |
---|---|---|---|
Before treatment | 0 | 50 | 276 |
After treatment | 180 | 50 | 126 |
4. Conclusion
The magnetic composite of ZnO and chitosan was successfully synthesized by a combination of sol-gel and co-precipitate methods. Structure, morphology and magnetic properties of the prepared composite were characterized by modern physicochemical methods such as IR, XRD, SEM, TGA and VSM. The degradation of RB198 was affected by the pH of the dye solution, illumination time, amount of composite and initial dye concentration. Experimental results showed that the percentage of photodegradation of ZnO/Fe3O4/CS reached above 70% after three times of recycling. Finally, the prepared catalyst exhibited as a potential material in environmental treatment with lower COD in comparison with untreated sample.
Acknowledgments
The authors would like to thank Industrial University in Ho Chi Minh City for financial support and Thanh Cong textile and dying company for dye donation.