Abstract
Background & Aims. Increased nitric oxide is involved in abnormal hemodynamic parameters and respiratory function of cirrhotic patients. We aimed to quantify partitioning exhaled nitric oxide measurements in exhaled air in liver transplantation (LT) candidates and evaluate their relationships with chronotropic incompetence and aerobic capacity. Methods. We compared exhaled nitric oxide (NO) measurements, heart rate response and peak oxygen uptake during incremental exercise in liver transplantation candidates to those of controls. Results. As opposed to healthy control subjects, LT candidates displayed elevated alveolar NO, blunted chronotropic response and reduced V'O2 at maximal exercise. In LT candidates, median peak V'O2 was 18.7 ml kg−1 min−1 (interquartile range (IQR) 16.2; 21.8), corresponding to 65% (IQR 57; 72) of the predicted value. Compared with controls, LT candidates had increased levels of alveolar NO (median (IQR) 2.0 (1.2; 2.2) versus 3.1 (2.3; 4.5), p < 0.001). In controls, no relations were found between alveolar NO and V'O2 peak or heart rate reserve whereas in cirrhotic patients, negative correlations and negative slopes were found between alveolar NO and V'O2 peak and heart rate reserve decrease. Conclusions. Increasing alveolar NO could be a specific pathophysiological condition limiting aerobic capacity in LT candidates.
Export citation and abstract BibTeX RIS
Abbreviations
CANO | Concentration of NO in the gas phase of the alveolar or acinar region |
CI | chronotropic incompetence |
DL,CO | carbon monoxide diffusing capacity |
DawNO | Airway compartiment diffusing capacity of NO from the airway wall to the gas stream |
FENO | nitric oxide fraction in the exhaled air |
FEV1 | forced expiratory volume in first second |
FVC | forced vital capacity |
JawNO | Total flux of NO in the conducting airway compartment |
LT | liver transplantation |
LVEF | left ventricle ejection fraction |
MAP | mean arterial pressure |
MELD | Model for End-stage Liver Disease |
NO | nitric oxide |
PAPs | pulmonary artery systolic pressure |
TLC | total lung capacity |
V'E | minute ventilation oxygen uptake |
V'O2 | oxygen uptake |
V'CO2 | carbon dioxide output |
Introduction
Liver transplantation (LT) candidates experience major exercise intolerance that reflects patient disability and determines poor outcome [1]. We and other groups have proposed that objective quantification of peak exercise oxygen uptake (peak V'O2) can predict survival and morbidity both before and after LT [2–8]. Whereas muscle weakness, sarcopenia, and anemia contribute to exercise intolerance in patients with end-stage liver cirrhosis [1], cardiovascular function remains the major limiting factor of maximal V'O2 [9–11]. In cirrhotic patients, cardiovascular dysfunction is typically characterized by a hyperkinetic state associated with subclinical cardiomyopathy [9–11]. Along with these perturbations, a contributing factor of poor aerobic capacity is the limitation of cardiac output to increase due to chronotropic incompetence, which has been broadly defined as the inability of the heart to increase its rate commensurate with increased activity or demand [9, 12–14]. In cirrhotic patients, chronotropic incompetence has long been recognized and attributed to impaired β-adrenoceptor signaling pathways, blunted muscarinic responsiveness and altered membrane fluidity affecting receptor ligand interactions [12, 13].
In cirrhotic patients, reduced nitric oxide (NO) bioavailability in the hepatic microcirculation contrasts with increased endothelial NO production in the extra-hepatic circulation [11–13]. Elevated cardiac output and vasodilation in pulmonary and systemic circulation have been related to increased NO levels [14, 15]. In addition, NO may also contribute to blunted chronotropic response to catecholamines, mechanisms of which have been related to β-adrenergic receptor function inhibition and abnormal autonomic cardiac regulation [16, 17]. As a biomarker, bulk exhaled NO has been validated for chronic inflammatory airway disorders, as well as endothelial dysfunction of the pulmonary circulation [18]. Multiple exhalation flow rate methods have been described for partitioning bulk exhaled NO measurements into alveolar and large airway components, i.e. alveolar NO concentration (CANO) and bronchial production, respectively [19–21]. Interestingly, previous studies in cirrhotic patients suggest that CANO may reflect endothelial NO production of the pulmonary and systemic circulation [22–24]. Consistently, inhibition of NO synthesis by nebulized N(G)-Nitro-L-arginine methyl ester (L-NAME) in patients with hepatopulmonary syndrome reduced cardiac index decrease and increased pulmonary and systemic vascular resistance [25]. It was hence proposed that CANO actually reflects overall endothelial NO production, which may be increased in both pulmonary and systemic vasculature of cirrhotic patients.
The aims of the present study were therefore to quantify partitioning exhaled NO measurements in exhaled air and their relations to chronotropic incompetence and low levels of aerobic capacity (peak V'O2) in end-stage cirrhotic patients.
Patients and methods
Patients and healthy subjects
This study was conducted in accordance with the French legislation on non-interventional studies and was approved by the Ethical Committee under number HP1824.
Patients were evaluated by our medical team, directed by surgeons, anesthesiologist and hepatologists, and considered potentially suitable for LT. All consecutive LT candidates were included. We excluded patients transplanted with national priority in an emergency setting (acute liver failure/fulminant hepatitis without underlying cirrhosis) and those who underwent multi-organ transplantation or liver retransplantation. Patients were not included in this series if they had primary cardiac diseases or moderate-to-severe porto-pulmonary hypertension. LT candidates had no history of atopy and were free from bronchial asthma, allergies, pulmonary tuberculosis, or recent respiratory tract infection. From the database of our institution, we prospectively identified and reviewed charts of all consecutive patients.
Healthy control subjects were receiving no medication and had no evidence of cardiopulmonary or allergic disease at the time of the study. The subjects had normal ventilatory function parameters, they had no respiratory symptoms or infections in the preceding four weeks and they were not taking any drug that could affect functional tests.
Clinical and cardiovascular evaluation
Clinical information about comorbidities and medical history were recorded. Individual patient records included medical conditions, biological parameters, Child−Turcotte−Pugh score (CHILD) and Model for End-Stage Liver Disease (MELD, calculated from serum bilirubin, INR, serum creatinine levels) score. Transthoracic echocardiography was systematically performed among LT candidates in the preoperative period according to the American Society of Echocardiography guidelines. If pulmonary artery systolic pressure (PAPs) was elevated, right side heart catheterization was performed. Patients with pre-existing severe pre-capillary pulmonary hypertension (mean pulmonary artery pressure >35 mmHg) associated with severe right ventricular dysfunction or high levels of pulmonary vascular resistance were excluded from this study.
Pulmonary function
Standard forced expiratory spirometry (forced expiratory volume in the first second (FEV1) and forced expiratory vital capacity (FVC)), body plethysmography total lung capacity (TLC)), and diffusing capacity of the lung for carbon monoxide (DL,CO) were performed (MasterScreen™ body plethysmograph, Viasys, France) using European Respiratory Society and American Thoracic Society guidelines.
Cardiopulmonary testing
Cardiopulmonary exercise testing was performed according to standardized procedures using an electromagnetic braked cycle ergometer. Exercise protocol involved an initial 2 min of rest, followed by unloaded cycling for 2 min then a progressively intensity increment every minute (10 W min−1) until exhaustion at a pedaling frequency of 60–65 rpm. Subjects were continuously monitored using 12-lead ECG (Cardiosoft, CareFusion, France). Blood pressure assessed by sphygmomanometry were recorded every 2 min. Subjects respired through an oro-nasal mask (Hans Rudolf 7450 SeriesV2™ Mask, CareFusion, France).
Breath-by-breath cardiopulmonary data (Vyntus, CareFusion, France) were measured at rest, warm up and incremental exercise testing. Before each test, oxygen (O2) and carbon dioxide (CO2) analyzers and flow mass sensor were calibrated using available precision gas mixture and a 3-L syringe, respectively. Minute ventilation (V'E), oxygen uptake (V'O2), carbon dioxide output (V'CO2) were recorded as concurrent 10 s moving averages, as was ventilation anaerobic threshold (AT) determined by the V-slope method. Ventilatory reserve was calculated as (MVV—peak V'E)/MVV* 100, where MVV is maximal voluntary ventilation estimated as FEV1 multiplied by 35. Peak values were averaged over the last 30 s of exercise. Patient effort was considered to be maximal if two of the following occurred: predicted maximal work is achieved, predicted maximal heart rate (HR) is achieved, V'E/V'O2 > 45, lactate level >6 mmol L−1, RER >1.10 and pH drop >0.06, as recommended by the ATS/ACCP. During the study period, mean values between qualified replicate tests performed weekly on control subjects were 5.1 ± 4.2%, 5.4 ± 3.2%, 6.1 ± 2.2% for peak V'O2, V'CO2 and V'E respectively. The percentage of heart rate reserve used at peak exercise was referred to [(HRstage − HRrest)/(220−age in years −HRrest)] * 100, where HR is heart rate.
Measurement of NO concentrations in exhaled air
All subjects abstained from food and coffee for 2 h prior to performing Pulmonary Function Tests, and were asked to not participate in strenuous activity for 1 h prior to fraction of exhaled NO (FENO) measurements. FENO levels were measured just before Pulmonary Function Tests with a chemiluminescence NO analyzer (NO analyzer CLD 88 Series Eco Medics AG, Switzerland) using the single-breath on-line method in accordance with the ATS recommendations. Subjects inhaled NO-free air to total lung capacity and exhaled against a pressure of 10–20 cm H2O into an exhalation circuit of the analyzer, which consisted of an ultrasonic flow meter, two-way valve and sampling port. Visual feedback on a computer monitor helped the subjects to maintain exhalation at four expiratory flow rates of 30, 50, 100 and 300 ml s−1. NO concentrations were measured during controlled expiration at the first stable FENO plateau for at least three seconds (FENO variations <10% or 1 ppb). Two measurements at each flow were required. If the FENO values differed by more than 10% (1 ppb for values less than 10 ppb), the patient performed a third exhalation. was recorded as the mean of two FENO measurements at 50 ml s−1 expiratory flow.
The nonlinear least squares method was used to estimate parameters of equation (1) directly, using data from all flow rates. This nonlinear equation, proposed by Horvath et al, gives additional information on the proximal airway NO output characteristics, alveolar NO concentration (), maximum airway flux (CawNO) and airway tissue diffusing capacity (
) [26].
was calculated according to equation (2):
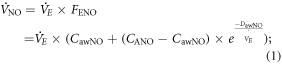

Parameters of the model were calculated by using the solver function in Excel 2010 software (Microsoft; Redmond, WA) to minimize the sum of the square residual values for Moreover, for each subject, pseudo coefficient of determination (pseudo R2) and Akaike information criterion for finite sample size (AICc) values were calculated to estimate the fit goodness [27].
Statistical analysis
Results are presented as median and interquartile ranges (IQR). Descriptive statistics with tests of normality (Shapiro−Wilk test) for quantitative variables were used. Two independent groups of data were compared by Wilcoxon's tests. Kruskal–Wallis tests were used to test differences between three groups, and post hoc comparisons were determined by Wilcoxon test with Bonferroni correction. Comparisons for categorical variables were performed with the Chi-squared test (with Fisher correction as appropriate). As values, but not CANO, are typically not normally distributed, data were log transformed prior to analysis. Relationships between quantitative variables were assessed by the Spearman's correlation coefficient (r).
To analyze the association between NO-derived parameters and peak VO2 or HR reserve, we used generalized linear model (GLM) adjusting for potential confounding factors: either age, body mass index (BMI), FEV1, current smoking, DLCO and β-blockers to patients or age, current smoking, BMI and FEV1 to controls. To estimate the cut-off level of CANO, ROC curve analyses were implemented with a threshold value of 20 ml kg−1 min−1 for the peak V'O2. The optimal cut-off for the ROC curves was defined as corresponding to the maximum value of Youden's index (sensitivity + specificity −1). Level of statistical significance was set at a p value <0.05. All statistical analyses were performed with SAS 9.4 software (SAS Institute Inc. Cary, USA).
Results
Characteristics of study population
We prospectively included 19 controls and 66 unselected consecutive patients with end-stage liver disease referred for preliminary evaluation for LT. Main characteristics of controls and cirrhotic patients are provided in table 1. Seventy percent of LT candidates had alcoholic cirrhosis. Viral cirrhosis was recorded in 12% of cases. Median MELD score was 12 (interquartile 8; 19). Median CHILD score was 7 (interquartile 5; 10). Patients were current smokers in 30% of cases versus 16% among controls. History of diabetes, systemic hypertension and COPD was recorded in 27%, 24% and 29% of patients, respectively. Pulmonary function was not different between the groups, due to a low grade of airway obstruction in COPD subjects (12 of the 18 subjects had a FEV1/FVC ratio between 65 and 70%). Controls and LT candidates had no history of atopy and were free from bronchial asthma, allergies, pulmonary tuberculosis and recent respiratory tract infection. Twenty seven (27%) of the patients were taking a β-blocker at the time of inclusion.
Table 1. Subject characteristics.
Controls | LT candidates | ||
---|---|---|---|
Sample size | n = 19 | n = 66 | p |
Age, year | 49.3 (29; 55) | 57.0 (51.0; 61.0) | <0.001 |
BMI, kg m−2 | 26.8 (21.9; 29.6) | 25.9 (23.5; 29.4) | 0.736 |
Gender, M/F | 16/4 | 55/11 | 0.743 |
Smoking status current/non-current | 3/16 | 20/46 | 0.255 |
Lung function test | |||
FEV1, % predicted | 102.0 (87.6; 106.9) | 97.4 (84.2; 108.2) | 0.625 |
FVC, % predicted | 103.6 (100.5; 113.0) | 104.2 (96.4; 114.4) | 0.724 |
FEV1/FVC, % predicted | 96.7 (84.8; 104.5) | 96.2 (90.1; 103.0) | 0.870 |
TLC, % predicted | 103.0 (98.2;112.0) | 101.0 (91.7; 108.2) | 0.564 |
DL,CO, % predicted | 83.0 (75.0; 96.2) | 69.0 (62.0; 76.0) | 0.017 |
Peak aerobic capacity | |||
Workload, W | 166 (143; 200) | 95 (85; 107) | <0.001 |
V'O2, ml kg−1 min−1 | 29.2 (21.3; 33.0) | 18.7 (16.2; 21.8) | <0.001 |
V'O2, % predicted | 92.8 (82.8; 109.0) | 65.0 (57; 72) | <0.001 |
V'E, l min−1 | 89.0 (79.0; 105.0) | 61.0 (55; 73) | <0.001 |
RER | 1.15 (1.12; 1.23) | 1.19 (1.11; 1.27) | 0.488 |
O2 pulse ml beat−1 | 14.5 (11.9; 17.0) | 12.7 (10.5; 14.9) | 0.029 |
HR, beat min−1 | 161 (141; 179) | 119 (101; 143) | <0.001 |
HR, % predicted | 94.7 (81.6; 101.1) | 69.3 (57.0; 82.0) | <0.001 |
HR reserve use, % | 89.6 (63.7; 101.0) | 49.8 (36.5; 68.0) | <0.001 |
Exhaled NO parameters | |||
![]() |
17.9 (10.8; 29.5) | 19.3 (13.8; 29.9) | 0.322 |
CANO, ppb | 2.0 (1.2; 2.2) | 3.1 (2.3; 4.5) | <0.001 |
JawNO, nl s−1 | 0.944 (0.512; 1.485) | 0.938 (0.621; 1.430) | 0.565 |
DawNO, pl s−1 ppb−1 | 9.9 (7.8; 13.5) | 10.1 (5.8; 15.2) | 0.936 |
adj R2 | 0.94 (0.79; 0.98) | 0.94 (0.88; 0.97) | 0.873 |
AICc | 75.5 (63.1; 80.9) | 78.8 (73.7; 85.4) | 0.014 |
Data are presented as median (interquartile range); BMI: body mass index; FEV1: forced expiratory volume in the first second; FVC: forced expiratory vital capacity; TLC: total lung capacity; DL,CO: diffusing capacity of the lung for carbon monoxide; V'O2: peak oxygen uptake; RER: respiratory exchange ratio; V'E: minute ventilation; HR: heart rate; NO: nitric oxide; fraction of exhaled NO at 50 mls−1 expiratory flow; CANO: alveolar NO concentration, JawNO: maximum airway flux; DawNO: airway tissue diffusing capacity; adj R2: adjusted R2, AICc: Akaike information criterion with correction for finite sample sizes. l: liter; nl: nanoliter; pl: picoliter; ppb is parts-per-billion. Bold values represent the significant results (Wilcoxon or Chi-square test).
Evaluation of cardiac and pulmonary function
Cardiopulmonary tests were analyzable in all patients who constituted the study cohort. Assessment of left ventricular LV function, valvular pathology, and pulmonary hypertension was performed among LT candidates by means of echocardiography. Left ventricular function was preserved in all LT candidates, as evidenced by an LV ejection fraction of 67 ± 3.5%. Median pulmonary artery systolic pressure was 28 mmHg (interquartile 23; 33). Pulmonary function assessment in LT candidates showed that median FEV1 and FEV1/FVC were within the normal predicted values. Compared with controls, LT candidates had reduced diffusing capacity of the lung for carbon monoxide (DL,CO).
Median peak VO2 was 18.7 ml kg−1 min−1 (interquartile 16.2; 21.8), corresponding to 65% (IQR 57; 72) of the predicted value. Compared with controls, LT candidates had reduced age-predicated maximal heart rate, heart rate reserve (table 1). We found the same results in patients taking β-blockers or not (data not shown). Effort was considered to be maximal in all patients and Borg-perceived exertion for respiratory and leg discomfort were scored 'hard' to 'very hard'. A significant correlation was found between peak V'O2 and BMI (table 2). Among controls, we found a significant correlation between peak VO2 and age (data not shown).
Table 2. Spearman correlation coefficient values between peak oxygen uptake, heart rate reserve use or NO-derived parameters and respiratory functional parameters among LT candidates.
Peak V'O2 | HRR | FENO50 | CANO | JawNO | DawNO | |
---|---|---|---|---|---|---|
Age | r = −0.15 | r = −0.01 | r = 0.30 | r = 0.24 | r = 0.28 | r = 0.13 |
p = 0.232 | p = 0.931 | p = 0.014 | p = 0.049 | p = 0.023 | p = 0.302 | |
BMI | r = −0.40 | r = −0.16 | r = 0.15 | r = 0.24 | r = 0.13 | r = 0.07 |
p < 0.001 | p = 0.203 | p = 0.243 | p = 0.052 | p = 0.305 | p = 0.567 | |
FEV1 | r = 0.14 | r = 0.11 | r = 0.21 | r = 0.21 | r = 0.22 | r = 0.02 |
% predicted | p = 0.275 | p = 0.385 | p = 0.084 | p = 0.088 | p = 0.067 | p = 0.850 |
FVC | r = 0.05 | r = 0.15 | r = 0.26 | r = 0.23 | r = 0.26 | r = 0.10 |
% predicted | p = 0.673 | p = 0.229 | p = 0.032 | p = 0.060 | p = 0.037 | p = 0.411 |
FEV1/FVC | r = 0.03 | r = −0.03 | r = −0.07 | r = 0.10 | r = −0.03 | r = −0.04 |
% predicted | p = 0.804 | p = 0.819 | p = 0.591 | p = 0.426 | p = 0.790 | p = 0.765 |
TLC | r = −0.03 | r = 0.22 | r = 0.02 | r = 0.11 | r = −0.04 | r = 0.02 |
% predicted | p = 0.782 | p = 0.072 | p = 0.896 | p = 0.395 | p = 0.759 | p = 0.872 |
DL,CO | r = 0.23 | r = 0.24 | r = 0.07 | r = −0.19 | r = 0.06 | r = −0.16 |
% predicted | p = 0.067 | p = 0.052 | p = 0.595 | p = 0.134 | p = 0.653 | p = 0.187 |
BMI: body mass index; FEV1: forced expiratory volume in the first second; FVC: forced expiratory vital capacity; TLC: total lung capacity; DL,CO: diffusing capacity of the lung for carbon monoxide; V'O2: peak oxygen uptake; HRR: Heart rate reserve use; fraction of exhaled NO at 50 ml s−1 expiratory flow; CANO: alveolar NO concentration; JawNO: maximum airway flux; DawNO: airway tissue diffusing capacity.
NO-derived parameters
CANO levels are significantly higher in LT candidates than in controls (table 1), and this difference remains after adjusting for age and smoking status (p = 0.014). Tables E1 in supplementary material available online at stacks.iop.org/JBR/12/046008/mmedia shows NO measurements in LT candidates and controls according to tobacco status. Among patients, current smokers had, compared with non-current-smokers, lower FENO values for four exhalation flows. Among controls we had observed same trends but only FENO for 30 and 50 ml s−1 were statistically lower in current smokers.
We found a significant correlation between CANO, JawNO and age, and between
JawNO and FVC among LT candidates. Correlation between CANO and FEV1 or FVC was close to the significance (table 2). Among controls, we found a significant correlation between CANO and FVC. Correlation between CANO and FEV1 or BMI was close to the significance (data not shown). Therefore, the following factors have been introduced in multivariate analyses: age, BMI, FEV1, current smoking, DL,CO and β-blocker for the cirrhotic patients.
Whereas controls and patients had similar levels, LT candidates had increased levels of alveolar NO, CANO (table 1). Adjusted coefficient of determination was not statistically different between patients and controls; AICc was slightly low in both controls and patients (table 1). This means that the fit goodness of the nonlinear model is similar between controls and patients. Thus, the three parameters (CANO, JawNO and DawNO) had been evaluated under the same conditions.
CANO and relationship with V'O2 peak and heart rate reserve
We did not find any relation between NO-derived parameters and V'O2 peak or heart rate reserve in controls, whereas we found negative correlations and negative slopes between CANO and V'O2 peak and heart rate reserve decrease in LT candidates (figures 1 and 2, table 3). Moreover, no correlation was found between and V'O2 peak or heart rate reserve (table 3). Among patients, factors such as age, BMI, FEV1, current smoking, DL,CO and β-blocker had no confounding effects on CANO levels (table 3), in contrast to heart rate reserve.
Figure 1. Scatter plot of peak V'O2 versus alveolar nitric oxide (CANO) in LT candidates. The Spearman correlation coefficient was used to test the correlation (r = −0.41, p < 0.001).
Download figure:
Standard image High-resolution imageFigure 2. Scatter plot of heart rate reserve use versus alveolar nitric oxide (CANO) in LT candidates. The Spearman correlation coefficient was used to test the correlation (r = −0.28, p = 0.023).
Download figure:
Standard image High-resolution imageTable 3. Coefficients of linear regression between peak oxygen uptake, heart rate reserve use and each NO-derived parameter.
Controls | LT candidates | |||
---|---|---|---|---|
β (SE) | adjusted β (SE) | β (SE) | adjusted β (SE) | |
Outcome: Peak V'O2 | ||||
Log (![]() |
0.95 (2.95) | 2.68 (3.16) | −0.18 (0.96) | 0.82 (0.97) |
CANO ; ppb | 1.34 (2.54) | 1.18 (3.62) | −0.82 (0.29)** | −0.79 (0.31)* |
JawNO; nl s−1 | 2.3×10−6 (3.3×10−6) | 5.1×10−6 (3.5×10−6) | −0.01×10−6 (0.9×10−6) | 0.4×10−6 (0.9×10−6) |
DawNO; pl s−1 ppb−1 | 0.306 (0.386) | 0.127 (0.382) | −0.180 (0.078)* | −0.141 (0.077) |
Outcome: HRR | ||||
Log (![]() |
6.09 (7.75) | −0.45 (7.70) | −7.77 (4.99) | −8.12 (5.03) |
CANO ; ppb | −3.22 (6.79) | −9.80 (8.18) | −3.24 (1.58)* | −1.55 (1.73) |
JawNO; nl s−1 | 8.8×10−6 (8.7×10−6) | 2.2×10−6 (9.0×10−6) | −3.9×10−6 (4.9×10−6) | −5.7×10−6 (4.9×10−6) |
DawNO pl s−1 ppb−1 | −0.099 (1.047) | −0.266 (0.908) | −0.742 (0.418) | −0.331 (0.414) |
V'O2: peak oxygen uptake; HRR Heart rate reserve use; fraction of exhaled NO; CANO: Alveolar NO concentration; JawNO: maximum airway flux of NO; DawNO: airway tissue diffusion capacity of NO. β coefficient of linear regression, SE : standard error; adjusted β coefficient of multi-linear regression after adjustment for on age, BMI, FEV1, current smoking, DL,CO and β-blocker to patients or after adjustment for age, current smoking, BMI, and FEV1 to controls: *p < 0.05, **p < 0.01.
The results of ROC curve analyses showed that area under the curve (AUCs) was 0.713 [0.584; 0.842] with an optimal CANO cut-off of 2.4 ppb which refers to a peak V'O2 value lower than 20 ml kg−1 min−1.
Discussion
Our study performed in end-stage cirrhotic patients investigated whether alveolar NO (CANO) increase was associated with reduced V'O2 peak, limiting convective O2 transport to skeletal muscle and aerobic capacity. As opposed to control healthy subjects, LT candidates displayed elevated CANO, blunted chronotropic response and reduced V'O2 at maximal exercise.
Whereas increased NO production is implicated in many aspects of the abnormal cardiovascular profile observed in cirrhotic patients [11–13], direct measurements of NO remain challenging. Alternatively, non-invasive measurement of NO in the exhaled air has been proposed to reflect endothelial bioavailability of NO in cirrhotic patients [22–24]. In humans, three forms of NO synthase, viz. endothelial, inducible and neurogenic NO synthases, are expressed and may be responsible for synthesis of NO that is measured in exhaled breath [18]. Development of mathematical models to interpret flow-dependent physiology of NO dynamics have been used to partition alveolar NO concentration from central airway NO production. Numerous statistical methods have been developed to estimate an individual's two-compartment model NO parameters from measurements of fractional concentration of exhaled NO at multiple flow rates [19–21], with different methods producing different estimates [28]. The three estimated parameters (CANO, JawNO and DawNO) are interpreted as providing information on proximal and distal NO sources. Unfortunately, we were not able to apply the recent recommendations of Horvath et al because we had already started recruiting our patients. Therefore, we have used a method that evaluates two-compartment model NO parameters from the four flow rates (30, 50, 100 and 300 ml s−1).
We found a significant correlation between CANO and age, as already shown by Högman et al [29], and we also found that the controls in our study were younger than the LT candidates, so that the higher levels of CANO observed in LT candidates could be explained by their age. However, the higher levels of CANO in LT candidates remain significantly different from those of controls after adjusting for age.
Smoking habits are known to decrease the production of NO. Malinovschi et al found lower CANO and levels in current smokers than in never-smokers. Interestingly, they also found that the levels of CANO among ex-smokers were not different from those of non-smokers and higher for subjects exposed to second-hand smoke [30]. In our study, we found that smoking has decreased
and JawNO values in LT candidates and controls whereas CANO values were not different between groups. It would have been interesting to know the proportion of ex-smokers in our study population but unfortunately, smoking status has been coded according to a state of current smoker or not current smoker. We did not collect the status of 'former smoker'.
Although and JawNO levels were not different between patients and controls, we found higher CANO levels in LT candidates. Our ranges of CANO levels in controls and in cirrhotic patients are lower than those reported by Delclaux et al [24], but CANO levels in our controls are similar to those found by Lázár et al [31].
Proposed mechanisms of increased CANO levels in liver cirrhosis include increased alveolar production of NO together with an impairment of diffusion across the alveolar capillary wall or increased excretion of NO from the pulmonary circulation into the alveolar [22–24]. The first hypothesis could be retained due to DL,CO impairment of LT candidates. In our study, adjustment for DL,CO did not modify CANO levels, which remained higher in the LT candidates compared with controls (data not shown). As an alternative concept, elevated CANO in liver cirrhosis may be explained by increased NO production by the pulmonary and systemic vascular endothelium [22, 23]. Indeed, experimental studies have shown that hepatopulmonary syndrome in rats is related to increased pulmonary NO production dependent primarily on increases in expression and activities of inducible NO synthase within pulmonary vasculature [32]. In cirrhotic patients, increased NO is involved to the enhanced endothelium-mediated vasodilation and hyporeactivity to vasopressors in forearm vessels [33]. In line with these studies suggesting that NO production is increased in the pulmonary and systemic vasculature, CANO levels in cirrhotic patients correlated positively with elevated cardiac index and negatively with low systemic vascular resistance [22].
A possible meaning of increased CANO is that NO overproduction represents a specific pathophysiological condition limiting aerobic capacity [34]. Indeed, increased CANO represents a clinical trait of patients with end-stage liver cirrhosis. Our results show that CANO levels are significantly higher in LT candidates than in controls, and this difference remains after adjusting for factors that modify CANO values such as age or smoking status [29, 30]. Moreover, increased CANO in LT candidates, but not was associated with low V'O2 peak, suggesting poor cardiovascular conditions. The correlation between CANO and heart rate reserve further suggests that NO may interfere with regulation of heart rate, leading to chronotropic incompetence. This is consistent with well-known effects of increased NO production on the autonomic nervous system. In experimental studies, increased NO release participates to blunted chronotropic and inotropic responses to β-adrenergic stimulation through mechanisms involving cGMP-mediated inhibition of β-adrenergic receptor' function. NO may also directly perturb regulation of the cardiac autonomic nervous system [16, 17]. NO alters pre- and postganglionic sympathetic nerve activities, as well as vagal control, which contribute to bradycardic and abnormal chronotropic responses [35]. Overall, NO-induced abnormal heart rate regulation can explain, at least in part, our results showing a weak relationship between CANO and heart rate reserve use in the univariate model. However, we found that this relationship disappeared after adjustment for β-blockers, whereas the relationship between V'O2 peak and CANO remained.
Compared with healthy controls, LT candidates had lower median peak V'O2, which was less than 60% of predicted value in 36% of cases. We confirm that impaired aerobic capacity was associated with chronotropic incompetence as evidenced by low level of heart rate reserve utilization at submaximal exercise. Whereas blunted chronotropic response was associated with increased CANO levels, multivariate analysis failed to identify elevated CANO has an independent factor of chronotropic incompetence in LT candidates. This conclusion indicates that, beside chronotropic incompetence, alternative mechanisms can limit cardiovascular performance in cirrhotic patients. For example, radionuclide ventriculography and echocardiography have confirmed impaired left ventricular contractile dysfunction at submaximal exercise [36, 37]. Hence, subclinical cardiomyopathy in cirrhotic patients may limit cardiac output increase and aerobic capacity due to impaired LV stroke volume. Because cardiac contractile function is difficult to assess during exercise, so-called O2 pulse, i.e. V'O2 to heart rate ratio, has been extensively used as a non-invasive surrogate for stroke volume. We found that O2 pulse was markedly reduced in LT candidates compared with controls, suggesting impaired cardiac function. Unfortunately, confounding covariates—i.e. V'O2 and heart rate—included in the O2 pulse calculation made it inadequate for further analysis.
In conclusion, we have identified increased CANO levels in LT candidates as an independent factor in peak V'O2. Although blunted chronotropic response is a consistent finding in patients with cirrhosis, heart rate reserve use did not clearly correlate with CANO levels. These findings suggest a role of systemic NO in limiting aerobic capacity in cirrhotic patients via cardiac performance.
Author's contribution
HULO Sébastien (HS), EDME Jean Louis (EJL), INAMO Jocelyn (IJ), VAN BULCK Richard (VR), DHARANCY Sébastien (DS), NEVIERE Rémi (NR).
NR, HS, EJL: Authors make substantial contributions to conception and design, and/or acquisition of data, and/or analysis interpretation of data and writing up of the paper. Authors give final approval of the version to be submitted. VR: Author makes substantial contributions to data management. IJ, DS: Authors participate in drafting the article or revising it critically for important intellectual content. All authors give final approval of the version to be submitted.
Acknowledgments
All authors declare having no conflicts of interest. The study was funded by own funds of our institutions without external financial support. The authors thank all of the staff of the Pulmonary Functional Tests Department for their help in this work.