Abstract
This study utilized the freeze-drying method to create a chitosan (CS) and polyvinyl alcohol (PVA) sponge. To enhance its antibacterial properties, curcumin and nano silver (Cur@Ag) were added for synergistic antibacterial. After adding curcumin and nano silver, the mechanical properties of the composite sponge dressing (CS-PVA-Cur@Ag) were improved. The porosity of the composite sponge dressing was closed to 80%, which was helpful for drug release, and it had good water absorption and water retention rate. The nano silver diameter was 50–80 nm, which was optimal for killing bacteria. Antibacterial tests used Escherichia coli and Staphylococcus aureus demonstrated that little nano silver was required to eliminate bacteria. Finally, in the rat full-thickness skin wound model, the composite sponge dressing can promote wound healing in a short time. In summary, CS-PVA-Cur@Ag wound dressing could protect from bacterial infection and accelerate wound healing. Thus, it had high potential application value for wound dressing.
Export citation and abstract BibTeX RIS
1. Introduction
The human skin serves as the primary defense against physical, chemical, and pathogenic microorganisms, making it the largest organ of the body [1]. In cases of delayed healing, wounds can result in significant fluid loss and severe tissue necrosis, potentially leading to life-threatening illnesses [2]. As such, wound treatment is crucial, and wound dressings have gained widespread attention in recent years. An ideal wound dressing should possess excellent biocompatibility, antibacterial activity, water absorption and retention, biodegradability, and no cytotoxicity [3]. Various wound dressings have been developed using different materials, including sponges with large pores that can absorb water. However, moisture in sponges often promotes the growth of microorganisms [4]. To address this issue, this study introduces a composite sponge material with antibacterial properties.
The selection of materials for wound dressings is vast, and in this study, chitosan (CS) was chosen due to its exceptional antibacterial properties, oxygen permeability, and ability to promote tissue regeneration [5]. CS is derived from chitin, a natural polymer found in various sources such as shrimps, crabs, insects, fungi, and plants [6]. The biological origin of CS makes it highly biocompatible, with characteristics such as biodegradability, reproducibility, non-toxicity, and non-irritation [7]. These properties make CS a promising material for wound dressing applications.
Polyvinyl alcohol (PVA) was incorporated into sponge dressings to enhance their mechanical properties. The addition of CS and cross-linking of PVA further improved the mechanical properties of the sponge dressing [8]. PVA is a water-soluble polyhydroxy polymer with numerous hydroxyl groups on its molecular chain, which provide excellent water solubility, film-forming properties, biocompatibility, and good mechanical properties [9]. Moreover, PVA is biodegradable and does not cause pollution. The hydrogen bonds between the hydroxyl groups on PVA and the amino groups on CS can be strengthened by adding cross-linking agents to enhance the mechanical properties of the composite [10]. In this study, glutaraldehyde was used as a cross-linking agent, and glycerol was added as a plasticizer to improve the mechanical properties of the composite.
In order to enhance the antibacterial properties of sponge dressings, we aim to incorporate antibacterial ingredients. Nano-scale metal particles have been widely used in the field of antibacterial agents due to their large specific surface area, high surface activity, and strong adsorption capacity [11, 12]. Among them, nano silver has been found to possess significant antibacterial properties [13]. The antibacterial mechanisms of nano-silver mainly include direct destruction of cell walls and membranes, release of silver ions to damage bacteria, and catalytic sterilization. As illustrated in figure 1, the mechanisms involve: (1) destruction of cell membrane and wall; (2) catalytic sterilization, where nano-silver can catalyze water or oxygen to produce reactive oxygen species that exhibit strong oxidizing properties, damaging the cell membrane and inhibiting bacterial growth; and (3) release of silver ions that can bind to DNA, destroy DNA sequences, inhibit DNA synthesis and protein expression [14].
Figure 1. Antibacterial mechanism of silver nanoparticles.
Download figure:
Standard image High-resolution imageCurcumin is a natural non-toxic antioxidant, antibacterial, anticancer and anti-inflammatory drug [15]. Toxicity studies have found that curcumin is safe in animal models and has a very high safety tolerance in humans. The minimum toxicity is 800 mg, and the single daily oral dose can reach 12 000 mg [16]. Curcumin can inhibit a surface protein of Gram-positive bacteria, thereby inhibiting bacterial adsorption and inhibiting Gram-positive bacteria against host cells. Although curcumin has good antibacterial properties and high safety to human body, its poor water solubility and low utilization rate make it not widely used [17]. In this study, metal chelates were used to increase the solubility of curcumin, so that nano-silver and modified curcumin were synergistically antibacterial, and the antibacterial properties of CS-PVA composite sponge dressing were increased.
Currently, extensive research has been conducted on CS, PVA, and nano silver [18]. An example of this is the work of Anisha et al who developed a wound dressing sponge for diabetic feet using CS hyaluronic acid/nano silver. This sponge combines the broad-spectrum antibacterial potential of nano silver with the enhanced hemostatic properties of CS and the excellent moisturizing ability of hyaluronic acid to provide an optimal environment for wound healing [19]. Similarly, Shyna et al developed a cross-linked CS and PVA mixed dressing that can instantly absorb sweat and has good liquid storage capacity due to the formation of pores [20]. However, no research has investigated the use of CS, PVA, nano-silver and curcumin together. Our study combined the broad-spectrum antibacterial properties of nano-silver and curcumin, with the biocompatibility of CS, and the mechanical properties of PVA to provide an optimal environment for wound healing.
From figure 2, this study did basic performance characterization, cell experiments and animal experiments. The basic performance characterization included scanning electron microscopy (SEM), transmission electron microscopy (TEM), Fourier transform infrared spectroscopy (FTIR), thermogravimetric experiments (TGA), x-ray diffraction (XRD), UV-ray diffraction (UV), porosity, water vapor transmission rate, swelling rate, water retention rate and mechanical properties. The cell experiments included drug release, antibacterial performance and cytotoxicity. The animal experiments included wound healing test and histological examination. Curcumin and nano-silver antibacterial dressings had the following highlights. Compared with the CS and PVA composite sponge dressing, the mechanical properties of the composite sponge dressing with antibacterial components were improved. Good mechanical properties facilitate the replacement of sponge dressings during clinical use and prevent adhesions from being easy to clean. The minimum inhibitory concentration of synergistic antibacterial curcumin and nano silver decreased, indicating that the antibacterial ability of curcumin and nano silver was improved. The antibacterial and anti-inflammatory effects of curcumin and nano-silver promoted wound healing, and the synergistic antibacterial composite sponge dressing could promote wound healing in a short time.
Figure 2. Highlights of the article structure.
Download figure:
Standard image High-resolution image2. Methods
2.1. Materials
The following reagents were obtained from Aladdin Reagent Co. Ltd: CS (medium viscosity 200–400 mPa·s), PVA, glutaraldehyde (50%), glycerin, tannic acid, curcumin, silver nitrate solution (1%), ferric chloride hexahydrate, dimethyl sulfoxide; bovine serum albumin.
2.2. Preparation of compound sponge wound dressings
2.2.1. Preparation of CS/PVA solution
Two grams of CS were dissolved in a 1% acetic acid solution to yield a CS solution with a concentration of 2% (w/v). Simultaneously, 5 g of PVA were dissolved in distilled water to produce a PVA solution with a concentration of 5% (w/v). Subsequently, the CS and PVA solutions were combined in a volumetric ratio of 2:1 and mixed for a duration of 1 h.
2.2.2. Preparation of antibacterial liquid
Curcumin was dissolved in dimethyl sulfoxide to yield a 50 mg ml−1 solution, while tannic acid was separately dissolved in deionized water to produce a solution with a concentration of 50 mg ml−1. Additionally, FeCl3 was dissolved in deionized water to prepare a solution with a concentration of 5 mg ml−1. Subsequently, 0.1 ml of the curcumin solution was introduced to deionized water, followed by the addition of 0.1 ml of the tannic acid solution and 0.1 ml of the FeCl3 solution. Finally, various concentrations of silver nitrate solution were incorporated, specifically 0.001%, 0.002%, and 0.003%. The specific content is shown in table 1.
Table 1. The content of each component in the antibacterial agent (10 ml).
Sample | Curcumin (50 mg ml−1) | AgNO3 (1%) | Tannin (50 mg ml−1) | FeCl3 solution (5 mg ml−1) |
---|---|---|---|---|
Cur@Ag1 | 0.1 ml | 0.1 ml | 0.1 ml | 0.1 ml |
Cur@Ag2 | 0.1 ml | 0.2 ml | 0.1 ml | 0.1 ml |
Cur@Ag3 | 0.1 ml | 0.3 ml | 0.1 ml | 0.1 ml |
2.2.3. Preparation of antibacterial sponge dressing
From figure 3,the CS solution and the antibacterial solution were combined in a volumetric ratio of 1:10 and mixed for one hour. Subsequently, an equivalent volume of PVA solution was introduced into the mixture. The process was finalized by the addition of 1% glutaraldehyde and 5% glycerin, facilitating cross-linking over two hours. Following the cross-linking step, the resultant solution was poured into a mold and subjected to freezing at −20 °C for 24 h. This was followed by a freeze-drying process lasting 48 h, conducted in a freeze dryer. These sequential steps were implemented to achieve a structured composite material with desired properties, ensuring the formation of an optimal matrix for subsequent applications in wound dressing or related biomedical contexts.
Figure 3. Preparation of CS-PVA-Cur@Ag sponge dressing.
Download figure:
Standard image High-resolution image2.3. Characterization of the prepared wound dressing
The characterization of nAg was performed using both ultraviolet–visible spectrophotometry (UV-2100) and TEM (JEOLJEM-2100). The scanning electron microscope (SU8020) was utilized to observe the microscopic morphology of the composite sponge. Fourier infrared spectrometry (Nicolet iS5) was used to analyze the molecular structure of the sample and the interaction relationship between the components. The dehydrated and dried sample was ground into ultrafine powder using a mortar, and the potassium bromide tableting method was employed to scan the range of 4000–500 cm−1. The crystal structure of the sample was analyzed using an x-ray diffractometer (D/Max 2500) at room temperature, with XRD patterns in the range of 10°–90° detected at a rate of 2° min−1. Finally, the thermal stability of the sample was analyzed using a thermogravimetric analyzer (Switzerland METTLER) in the nitrogen range, with the temperature increased from room temperature to 600 °C at a constant rate of 10 °C min−1 while continuously measuring the weight loss of the sample.
2.4. Porosity
Since the prepared sponge has a large volume and a large porosity, the volume method can be used to test its porosity. Pour pure water into the measuring cylinder and record the scale corresponding to the liquid level as V1 (ml). Put the dry sponge into the measuring cylinder, so that the sponge is completely immersed in pure water, and record the scale corresponding to the liquid level as V2 (ml). Take out the sponge with tweezers. The liquid level will drop due to the water-absorbing nature of the sponge gap. Record the scale as V3 (ml). Among them, V2–V3 represents the total volume of the sponge, that is the sum of the entity volume and the void volume; V1–V3 represents the void volume of the sponge. The ratio of the void volume of the sponge to the total volume of the sponge is the porosity (P) of the sponge. The calculation formula is:
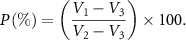
2.5. Water vapor transmission rate
Use a cylindrical plastic centrifuge tube slightly smaller than the diameter of the sponge as a test container, measure its inner diameter d with a vernier caliper, and its area is S (πd2/4). After pouring the same volume of deionized water into the plastic centrifuge tube, put the composite sponge into the nozzle of the plastic centrifuge tube to seal, make sure that the depth of each sponge entrance is 10 mm, and cut off the excess sample with a knife parallel to the nozzle. The initial mass of the system was weighed with an electronic balance as m0, then it was placed in a constant temperature incubator at 37 °C, and after time t, the system was taken out and its mass mt was measured. By measuring the quality change of the system and calculating the amount of water vaporized through the sponge, the water vapor transmission rate MVTR is obtained:
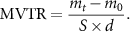
2.6. Swelling degree and water retention rate
Characterized by measuring its mass change in PBS buffer. Measure the initial mass as m1, soak in the PBS buffer solution, take out the samples at different times, wipe off the excess liquid on the surface with filter paper, and weigh the mass as m2. After weighing, add an equal amount of PBS solution until the end of the test. The calculation formula of swelling degree is:

The sample was soaked in pure water for 24 h to reach saturation, and its mass was called m3. The samples were taken out at different times, and the excess liquid on the surface was wiped off with filter paper. The mass was recorded as m4 by weighing. The calculation formula of water retention rate is:

2.7. Mechanical performance test
First, the composite sponge sample is cut into a standard sample of 50 × 20 × 5 mm, and then the sample is placed in a universal testing machine for tensile test. The test parameters are: the load is 50 N, and the tensile rate is a constant rate of 5 mm min−1. Each sample was repeated five times.
2.8. Drug release
The amount of drug released was analyzed with the aid of an ultraviolet spectrophotometer. First, the absorption spectrum containing curcumin and nano-silver antibacterial agent (Cur@Ag) is obtained through full-wavelength scanning and the largest characteristic absorption peak is found. Secondly, measure the absorbance of curcumin and nano-silver antibacterial agent (Cur@Ag) in different concentrations at the characteristic absorption peak, and establish a linear relationship between the absorbance of the solution and the concentration of the solution (according to Lambuber's law, the absorbance is between 0 and 1. At the time, the absorbance has a linear relationship with the concentration). Finally, the cumulative drug release rate can be obtained by converting the absorbance into a specific concentration and comparing it with the total drug load.
2.9. Antibacterial properties
The antibacterial properties of the composite sponge against Staphylococcus aureus (S. aureus) and Escherichia coli (E. coli) were studied, and antibacterial experiments were conducted using two methods.
Firstly, the zone of inhibition test method was employed. Silver sponges of different concentrations were soaked in PBS solution for 24 h to obtain extracts. E. coli and S. aureus were prepared into a bacterial suspension of 106CFU ml−1. Then, 0.1 ml of bacterial liquid was evenly smeared on the surface of the culture medium, and a 20 mm filter paper soaked in the extract was attached to the surface of the culture medium. Then petri dish was placed in a 37 °C incubator and incubated it upside down for 24 h. The size of each inhibition zone was measured after removal.
Secondly, samples of equal mass and different concentrations, along with 10 ml of LB medium, were placed into test tubes, including a blank control group. S. aureus and E. coli were added to the test tubes and diluted to 106 CFU ml−1. Then the test tubes were incubated in a 37 °C incubator for 6, 12, and 24 h, and the OD value at 630 nm was measured.
2.10. Cytotoxicity
First, the composite sponge was cultured in RPMI-1640 medium for 24 h to obtain the extract. After the cells were cultured in a 96-well plate for 12 h, the culture medium was removed, the extract and 10% fetal bovine serum were added. After culturing for 24 h and 48 h, the liquid was removed and MTT solution was added, the culture was continued for 4 h. Then terminate the culture, remove the MTT culture solution, add DMSO, place on a shaker to shake at low speed for 10 min to fully dissolve the crystals, and then measure the light absorption value with an enzyme-linked immunoassay at a wavelength of 490 nm,

2.11. Wound healing assay
This experiment uses 30 SD rats, provided by Lilai Company, and randomly divided into five groups, each with 6 rats. The first group was treated with gauze as a blank control group; the second group was a commercially available CS sponge; the remaining group was a composite sponge dressing containing curcumin and nano-silver with different concentrations. After the SD rats were anesthetized, the back hair was cut, and a 1.0 cm × 1.0 cm full-thickness skin wound model was established on the back; each group was treated accordingly, and the wound was covered with a wound dressing; the rats were placed in a single cage after the operation Feed them to prevent biting each other's wounds, and change their dressings every 3 d.
Wound healing is usually a complex process, including inflammation, proliferation and remodeling. In order to further understand the tissue regeneration of the wound in this study, HE and Masson tricolor staining were used for histological analysis on the 3rd, 7th, 10th, and 15th days respectively, and the wound healing was observed, and the wound healing area was used to characterize the wound. The formula is as follows (S0—initial wound area; S1—wound area after healing):

3. Results and discussion
3.1. Preparation of composite dressing
The preparation method for curcumin nanoparticles involved adding tannic acid to form a water-soluble film, which was not stable and easily damaged (figure 4(a)). To increase stability, metal ions were added for cross-linking, resulting in a formation of a TA-Fe chelate layer. Silver nitrate was then reduced to nano-silver particles through the reducibility of tannic acid. This method has several advantages, including simplicity, rapidity, increased water solubility of curcumin, and synergistic antibacterial effects between curcumin and nano silver.
Figure 4. (a) Schematic diagram of antibacterial preparation (b) UV spectrum of antibacterial agent (c) TEM of antibacterial agent.
Download figure:
Standard image High-resolution imageThe antimicrobial solution's color gradually darkened as the concentration of silver nitrate increased, and the ultraviolet–visible spectrum showed a maximum characteristic absorption peak at 430 nm, with corresponding absorbance increasing as silver nitrate content increased (figure 4(b)). The transmission electron microscope image of the antimicrobial agent revealed spherical nanoparticles with a diameter of about 20–80 nm (figure 4(c)), indicating better surface effect and small size effect for improved antibacterial properties [21].
3.2. Fourier transform infrared spectroscopy (FTIR)
The infrared spectrum analysis serves a dual purpose of identifying the specific functional groups present in the material and observing any changes in the position and intensity of the characteristic peaks, as well as detecting the emergence of new characteristic peaks to determine any chemical reactions. As shown in figures 5(a) and (b), the characteristic peaks of PVA include the absorption peak of O–H stretching vibration at 3279 cm−1, the absorption peak of aliphatic C–H stretching vibration at 2940 cm−1, the characteristic peak of C=O at 1716 cm−1, and the absorption peaks of C–C skeleton vibration at 1087 cm−1 and 1024 cm−1. In contrast, CS exhibits a broad peak at 3300 cm−1 that is a mixture of O–H and N–H stretching vibrations, an absorption peak at 2880 cm−1 for C–H stretching vibration, and peaks at 1537 cm−1 and 1405 cm−1 for C–H bending vibration, with an absorption peak of C–C skeleton vibration at 1065 cm−1 [22].
Figure 5. (a) FTIR for CS, PVA, CS-PVA (b) FTIR for CS-PVA-Cur@Ag1, CS-PVA-Cur@Ag2, CS-PVA-Cur@Ag3 (c) XRD (d) TGA.
Download figure:
Standard image High-resolution imageThe broad peak observed in the blend of CS-PVA at 3268 cm−1 represents the mixed absorption peak of O–H and N–H stretching vibrations. The addition of PVA caused a shift in the O–H and N–H stretching vibration mixing peaks from high wave number to low wave number, which can be attributed to the effect of hydrogen bonding factors on the functional groups, leading to peak shifting. With an increase in hydrogen bonding, the stretching vibration absorption spectrum shifted to lower wave numbers. The C–O–C stretching vibration absorption peak observed at 1035 cm−1 indicates the occurrence of a cross-linking reaction. After glutaraldehyde cross-linking, a C=N Schiff base absorption peak appeared at 1645 cm−1, indicating the formation of a uniform composite sponge of CS and PVA through cross-linking. The spectra of the CS-PVA-Cur@Ag composite showed no peak changes, indicating that the Cur@Ag and CS-PVA composite had no chemical effect and that the nanoparticles were simply superimposed on the composite sponge.
3.3. X-ray diffraction pattern (XRD)
In figure 5(c), the diffraction peaks of CS were observed at 10.5 °C, 18.5 °C, and 22.5 °C. The peak at 10.5 °C corresponds to the hydrated crystal structure of CS, and the peak at around 20 °C is attributed to its anhydrous crystal structure. PVA, on the other hand, exhibits a strong diffraction peak at 19.5 °C [22]. If there were no interactions between CS and PVA molecules or if the interaction was very weak, the XRD spectrum would show the diffraction patterns of CS and PVA as simple stacking in proportion, with separate crystalline regions in the mixed sponge. However, the XRD results showed that the diffraction peak of CS at 10.5 °C was weakened in the mixed sponge due to the addition of PVA, indicating that the interaction between CS and PVA disrupted the original crystal structure of CS, leading to a new molecular arrangement and combination. This finding is consistent with the results of FTIR analysis. The anhydrous crystallization peak of the mixed sponge at 20.5 °C was weakened due to glutaraldehyde cross-linking of CS and PVA, which affected the orderly arrangement of molecules and destroyed the crystal structure of CS.
3.4. Thermogravimetric analysis experiment (TGA)
The TGA curve in figure 5(d) indicates that there is no degradation point in the temperature range of 30 °C to approximately 165 °C, implying that the molecular structure and chemical composition of the CS-PVA and CS-PVA-Cur@Ag materials remain intact with no cracking in this range. This result demonstrates the excellent thermal stability of the composite materials within this temperature range. As the composite material has strong hygroscopicity, the presence of moisture in the material during the experiment is inevitable. Therefore, it can be inferred that the attenuation of mass during this stage is primarily due to the evaporation of moisture. The quality loss at this stage indicates the good hydrophilicity of this composite material. Upon removal of moisture, the thermal degradation characteristics of the material will be clearly visible on the TGA curve.
The TGA curves of all four samples exhibit three stages: the first stage is 30 °C–165 °C, which corresponds to the evaporation of water in the sample; the second stage is 165 °C–350 °C, representing the partial cleavage of the cross-linked bonds of PVA and CS; and the third stage is 350 °C–500 °C, corresponding to the cleavage of the remaining cross-links and the oxidative degradation of the two polymer macromolecular chain skeletons. The maximum degradation temperature in the second stage of the four samples is approximately 210 °C. The TGA demonstrates that the CS-PVA composite sponge possesses higher thermal stability. The cross-linking results in a three-dimensional network structure, and there are hydrogen bonds within or between molecules in the sponge. As a result, the thermal stability of the composite sponge is enhanced. According to the thermogravimetric experiment, the thermal stability of CS sponge was good. Within 200 °C, the material would not decompose due to high temperature.
3.5. Scanning electron microscope (SEM)
In this research, a CS-PVA-Cur@Ag porous sponge dressing was prepared through freeze-drying of the CS-PVA and Cur@Ag solution. Figure 6 illustrates that the composite sponge possesses a soft texture. The microscopic surface and cross-sectional morphologies reveal that all four materials have a dense pore structure with interpenetrating pores. However, since Cur@Ag is present in the nano-scale range, its existence cannot be observed. The pore sizes of the four materials are approximately 100–250 μm. SEM images of different dressings indicate that the addition of Cur@Ag influences the dressing structure. The pore size increases after incorporating nano silver. As the concentration of nano silver increases, the pore size also increases, which enhances the absorption of liquids.
Figure 6. SEM of the composite sponge, the first row is cross section and the last row is surface view. (a) CS-PVA, (b) CS-PVA-Cur@Ag1, (c) CS-PVA-Cur@Ag2, (d) CS-PVA-Cur@Ag3, the unit length is 500 μm.
Download figure:
Standard image High-resolution image3.6. Porosity and water vapor transmission rate
Porosity is a crucial factor in determining the water absorption and drug release of sponges [21]. The porosity of the composite sponge dressing, measured by the volume method, is presented in figure 7(a). The sponge dressing containing nano-silver has a porosity of 81%, whereas that of the CS-PVA sponge dressing is 70%. The increase in porosity may be attributed to the addition of Cur@Ag antibacterial liquid, which elevates the water content. High porosity is desirable for wound dressings, as it enables the absorption of more exudate [22]. The ideal wound dressing should possess good air permeability to facilitate the proliferation and growth of epidermal cells and fibroblasts, and to maintain a proper balance of oxygen and carbon dioxide on the wound surface. Figure 6(b) shows the relationship between the water vapor transmission rate of composite sponge dressings with different contents. Sponges with higher nano-silver content exhibit a greater water vapor transmission rate, which aligns with the porosity trend. The degree of crystallinity and density of the structure significantly influence the air permeability of the composite material [23]. Overall, the composite sponge exhibits excellent air permeability, with a water vapor transmission rate exceeding 350 g m−2 d−1.
Figure 7. (a) Porosity of sponge for CS-PVA, CS-PVA-Cur@Ag1, CS-PVA-Cur@Ag2, CS-PVA-Cur@Ag3. (b) Water vapor transmission rate of sponge for CS-PVA, CS-PVA-Cur@Ag1, CS-PVA-Cur@Ag2, CS-PVA-Cur@Ag3. (c) Swelling rate of sponge for CS-PVA-Cur@Ag1, CS-PVA-Cur@Ag2, CS-PVA-Cur@Ag3. (d) Water retention rate of sponge for CS-PVA, CS-PVA-Cur@Ag1, CS-PVA-Cur@Ag2, CS-PVA-Cur@Ag3.
Download figure:
Standard image High-resolution image3.7. Swelling rate and water retention rate
To prevent wound infections and promote rapid healing, a moist and sterile environment is essential. Therefore, effective antibacterial dressings should have good water absorption and moisturizing capabilities [24]. The porous network structure of the composite sponge dressing provides it with excellent swelling properties. This experiment investigated the influence of the sponge content on swelling performance, as shown in figure 7(c). All four sponges rapidly absorbed water within the first 10 min and expanded in volume. The swelling rate remained relatively stable during the following 150 min. The composite sponge dressing had strong liquid absorption ability and high swelling rate, which is beneficial for absorbing a large amount of exudate. The CS-PVA sponge dressing demonstrated good moisturizing properties. However, as the concentration of nano-silver increased, the water absorption rate gradually decreased due to the aggregation of nano-particles, which destroyed the network structure of the composite material. Figure 7(d) displays the water loss of the CS/PVA sponge within 150 min. In the first 10 min, the sponge lost a lot of water, and then it remained basically stable. At this point, the sponge mass was 40%–70% of the sponge mass in the saturated state. The higher the water absorption capacity of the sponge, the greater the water loss under the action of gravity, so the water absorption and water retention rate of the sponge exhibit an opposite trend. Overall, the composite sponge dressing possesses excellent swelling properties and can effectively absorb exudate, promoting wound healing in a moist environment.
3.8. Mechanical properties
Good mechanical properties are important for wound dressings to provide effective coverage for extended periods of time without causing discomfort or hindering movement [25]. To assess the effect of nano-silver on the mechanical properties of the sponge dressing, we evaluated its tensile properties. Table 2 summarizes the results of the tests, which included measurements of Young's modulus, tensile strength, and elongation at break. Compared to CS-PVA, CS-PVA-Cur@Ag showed higher tensile strength and elongation at break. Specifically, CS-PVA had a tensile strength of 1.42 ± 0.66 MPa and an elongation at break of 25.33 ± 1.37%. The increase in tensile strength and elongation at break may be attributed to the uniform dispersion of nano-silver in the polymer. However, as the concentration of nano-silver increased, the tensile strength initially increased and then decreased. This decrease in tensile strength may be due to the aggregation of nano-silver, which hinders the formation of the CS network structure and reduces the tensile properties and fluidity of the sample [26].
Table 2. Tensile properties of CS-PVA, CS-PVA-Cur@Ag1, CS-PVA-Cur@Ag2 and CS-PVA-Cur@Ag3. All values are expressed as the mean ± SD for each group (n = 5).
Material | Young's modulus (MPa) | Tensile strength (MPa) | Elongation at break (100%) |
---|---|---|---|
CS-PVA | 1.42 ± 0.66 | 1.57 ± 0.16 | 25.33 ± 1.37 |
CS-PVA-Cur@Ag1 | 2.18 ± 0.64 | 1.84 ± 0.31 | 28.38 ± 1.94 |
CS-PVA-Cur@Ag2 | 2.75 ± 0.38 | 2.21 ± 0.23 | 37.81 ± 2.47 |
CS-PVA-Cur@Ag3 | 2.43 ± 0.25 | 2.18 ± 0.62 | 34.15 ± 1.13 |
3.9. Drug release
The antibacterial agent release behavior of the composite sponge dressing was evaluated by following the procedure shown in figure 8(a). First, the prepared curcumin and nano silver (Cur@Ag) antibacterial agent were diluted in PBS solution to different concentrations, and the absorbance at a specific concentration was measured to obtain the antibacterial agent release standard curve in PBS buffer [27]. Then, the composite sponge dressing containing the antibacterial agent was soaked in PBS buffer, and a suitable amount of liquid was collected at different time points. The absorbance at 430 nm was measured, and the corresponding concentration on the release standard curve of the antibacterial agent in PBS buffer was determined to calculate the amount of released antibacterial agent [28].
Figure 8. (a) Release standard curve of antibacterial agent (Cur@Ag) containing curcumin and nano silver diluted in PBS solution (b) cumulative release curve of drug containing antibacterial agent composite dressing.
Download figure:
Standard image High-resolution imageThe release behavior of different contents of antibacterial agents is shown in figure 8(b), and it follows a similar pattern. The release rate of curcumin and nano-silver particles was higher in the first 3 h, gradually decreasing between 3 and 15 h, and reaching a stable rate after 20 h. According to research, a burst release of drugs within a certain range is effective for antibacterial purposes as it can kill bacteria before they reproduce. Meanwhile, sustained drug release can prevent drug aggregation and improve the antibacterial effect. The drug release rate of the CS-PVA composite sponge dressing containing nano-silver ranges from 50% to 70%, and as the nano-silver content increases, the release rate also increases. With an increase in the concentration of antibacterial agents, the cumulative release rate of the drug was found to be 26%, 32%, and 39%.
3.10. Antibacterial performance
The inhibition zones of the composite sponge dressings containing various concentrations of antibacterial agents were evaluated by the zone of inhibition experiment, as depicted in figure 9(a). Two types of bacteria, S. aureus and E. coli, were employed for the experiment. It can be observed that the inhibition zone of CS-PVA-Cur@Ag1 was small, and the zone was initially formed since the concentration of nano-silver was close to the minimum inhibitory concentration. However, as the concentration of nano-silver increased, the zone of inhibition became more prominent, and the diameter of the zone increased accordingly. Figure 9(b) shows the histogram of the inhibition zone diameter produced by different concentrations of nano-silver.
Figure 9. Inhibition zone graph of composite sponge dressing containing nano silver and curcumin (b) inhibition zone diameter histogram.
Download figure:
Standard image High-resolution imageBased on the results presented in figure 9(b), the diameter of the inhibition zone for E. coli ranged from 22.4 mm to 28.1 mm, while that for S. aureus ranged from 21.2 mm to 23.1 mm. The diameter of the inhibition zone increased as the concentration of the antibacterial agent increased. Notably, the same concentration of the antibacterial agent produced different inhibition zones for the two bacteria, indicating that nanosilver has varying antibacterial efficacy against S. aureus and E. coli. Specifically, the antibacterial agent showed stronger efficacy against E. coli as evidenced by the larger inhibition zone at the same concentration.
From figures 10(b) and (d), it is evident that the blank control culture medium is relatively turbid, whereas the solution in the composite sponge dressing of pure CS-PVA has reduced turbidity compared to the blank control. The culture medium in the composite sponge dressing containing the antibacterial agent is relatively transparent. Upon soaking, the nano silver and curcumin are released from the sponge for sterilization. Figures 10(a) and (b) depict the absorbance of bacteria at 630 nm after cultivation in the medium for varying periods of time. The higher the absorbance, the higher the bacterial content in the medium. Within 6 h, the sponge dressing containing nano silver and curcumin demonstrated significant inhibition of bacterial growth, as evidenced by the considerable difference in absorbance between the composite sponge dressing containing nano silver and curcumin and the blank control group. As time progressed, the difference in absorbance between the composite dressing containing nano-silver and curcumin and the blank control group became even more pronounced.
Figure 10. The optical density at 630 nm was measured after incubating the composite sponge dressing for 6, 12 and 24 h: (a) (b) S. aureus, (c) (d) E. coli, the picture was taken after 12 h incubation at 37 °C.
Download figure:
Standard image High-resolution imageThe mechanism of action for the antibacterial properties of nano silver is generally understood as follows: The nano silver particles penetrate the bacterial cell wall and interact with the sulfhydryl groups of the cell synthetase, resulting in the destruction of its activity. Consequently, the bacteria lose their ability to divide and multiply. The nano silver is then released from the dead bacteria, and this process of sterilization is repeated, leading to its antimicrobial effect [29].
3.11. Cytotoxicity
Figure 11 illustrates the growth of endothelial cells on three different groups: the blank group, the control sponge, and the experimental sponge containing nanoparticles. The absorbance value in this context reflects the relative number of cell growth, with higher values indicating better cell proliferation. The lower the toxicity of the material, the better the promotion effect [30, 31]. As shown in figure 11, the absorbance values of all three groups increase over time, and the cell viability rate of the CS-PVA group was greater than 100%. CS is a natural polymer material with excellent biological activity that can promote wound healing and skin tissue repair, which explains why the cell viability rate of the CS-PVA group was greater than 100% when compared to the blank control group. The significant difference analysis indicates that there is no significant difference between the blank group, CS-PVA, and the load dressing containing nanoparticles. This finding suggests that the sponge containing nanoparticles has no obvious toxicity to endothelial cells and has a positive effect on cell growth. The CS-PVA-Cur@Ag1 demonstrated significant antibacterial properties at low concentrations, without causing any apparent cytotoxicity.
Figure 11. Cell viability of endothelial cells on samples at 24 and 48 h.
Download figure:
Standard image High-resolution image3.12. Wound healing test
Figure 12 depicts the wound healing rate of different materials. As shown in figure 12(a), on the third day of wound healing, the healing rate of the control group with gauze and the sample treated with the composite sponge are notably different. The wound healing area of the synergistic antibacterial sponge dressing can reach approximately 40%, indicating that it can greatly promote the early healing of the wound. In contrast, the wound healing area of the rats treated with gauze is only about 15%, suggesting a poor healing effect. By the 7th day, the wound healing rate of rats increased, and the difference in the wound healing area between the control group and the experimental group using gauze decreased. After 15 d, the wound healing area of the rats treated with the composite sponge could reach about 95%, whereas that of the rats using gauze was about 85%. The faster wound healing effect of the nano-silver and curcumin synergistic antibacterial sponge dressings prepared in this study compared with commercially available CS sponge dressings could be due to curcumin's antibacterial and anti-inflammatory effects that promote wound healing. However, figure 12(a) shows that a small amount of sponge dressing adheres to the wound surface. Therefore, it is recommended to add a layer of anti-adhesive mucosa in subsequent applications.
Figure 12. (a) Wound images treated with different materials at different times (b) wound healing rate of SD rats treated with different materials at different time points.
Download figure:
Standard image High-resolution imageThe histological examination of wound healing progress is shown in figure 13(a) using Hematoxylin and Eosin (HE) staining. The figure indicates that on the third day, there was significant inflammation and necrosis in a large area of skin tissue. On the seventh day, inflammatory cells decreased, and new capillaries started to form. By the fifteenth day, the epidermis had formed a scab and thickened, creating new epithelial tissue. In contrast, on the fifteenth day, samples 1 and 2 (treated with gauze and commercially available CS sponge dressing, respectively) still had crusts on the epidermis, and the formed epithelial tissue was not uniform. However, samples 3 and 4, treated with the synergistic antibacterial composite sponge dressing, had uniform epithelial tissue, which was thicker than that of samples 1 and 2. These results suggest that the synergistic antibacterial composite sponge dressing promotes the formation of uniform epithelial tissue, which may contribute to faster wound healing compared to the other treatments.
Figure 13. (a) HE stained sections treated with different materials at different times for skin tissue necrosis
or scab skin
epidermis thickening
new capillary (b) wound healing rate of SD rats treated with different materials at different time points.
Download figure:
Standard image High-resolution imageThe staining method of Masson can be used to observe the condition of collagen fibers in tissue sections. In this method, collagen fibers were stained blue, while red blood cells were stained orange-red. Figure 13(b) displays the tissue sections on the 10th and 15th days of wound healing. All samples display a significant amount of collagen fibers, which constitute the primary component of the dermis layer, indicating that the wound is healing effectively. Furthermore, the red portion in the figure represents the new capillaries. Samples 3 and 4 exhibit a higher number of new capillaries, whereas the blank control group treated with gauze displays the least number of new capillaries.
4. Conclusion
The CS-PVA-Cur@Ag composite sponge dressing prepared by freeze-drying had good water absorption and thermal stability, which could meet the requirements of replacing wound dressings for 2–3 d. The elongation at break of the composite sponge was 25%–40%, and the tensile strength was 1.5–2.2 MPa. Compared with pure CS and PVA sponge, the composite sponge with antibacterial agent had better mechanical properties. The silver content of the composite sponge dressing prepared by this method was 0.001%, 0.002%, 0.003%. When nano-silver was used alone, the minimum inhibitory concentration of nano-silver was 0.01%. When synergistic antibacterial, the minimum inhibitory concentration of nano-silver was 0.001%, and the concentration was reduced by about 10 times, which significantly enhanced the antibacterial ability of curcumin and nano-silver. In the rat full-thickness skin wound model, the synergistic antibacterial composite sponge dressing could promote wound healing in a short time, and the wound healing area reached 95% after 15 d (85% in the gauze control group), which has a significant effect on wound healing. Therefore, the CS-PVA-Cur@Ag composite sponge dressing has potential application value in future wound healing.
Acknowledgments
This study was funded by Grants from the National Key R&D Program (2016YFC1101101) and Science and Technology Bureau of Chongqing Yuzhong District (20210170). JinFeng Laboratory, Chongqing, China (jfkyjf202203001). We are also thankful for the support from the Chongqing Engineering Laboratory in Vascular Implants, the Public Experiment Center of State Bioindustrial Base (Chongqing) and National '111 Project' Base (B0625).
Data availability statement
All data that support the findings of this study are included within the article (and any supplementary files).
Conflict of interest
The authors declare no competing financial interest.
Ethics information
All the animal Experiment Protocol comply with the relevant laws, regulations and standards concerning animal welfare ethics. This Project has been supervised and approved by Laboratory Animal Welfare and Ethics Committee of Chongqing University. IACUC Issue No. COU-IACUC-RE-202 306-001.