Abstract
We report on the calculations of the K-shell differential radiative recombination (RR) rate coefficients for very low, in the range of meV, relative electron–ion energies. The rate coefficients were derived for bare uranium ions colliding with free electrons both within the nonrelativistic dipole approximation and using fully relativistic calculations. We show that even for such low relative ion–electron energies, the differential rate coefficient reveals strong relativistic effects. We demonstrate that the measurements of the relative electron energy dependence of the RR rates represent a very sensitive tool for precise studies of the RR process and, in particular, for probing the fine details of the relativistic effects in the RR of ions with electrons. The results are discussed in the context of the first x-ray state-selective RR experiment performed for very low relative electron–ion energies.
Export citation and abstract BibTeX RIS
1. Introduction
Electron–ion recombination is a fundamental group of processes occurring in collisions of highly charged ions with electrons, which is very important in many areas of basic and applied physics. One of the processes is electron–ion radiative recombination (RR), being the time-reversed photoionization process, and consequently the cross sections for both processes are related by the principle of detailed balance. In the RR process, a free electron is captured into the bound state of an ion with emission of a photon, which for inner shells of heavy ions is in the x-ray energy range. Consequently, the x-rays emitted in the RR of heavy ions with electrons provide a unique possibility for studies of atomic structure and collision dynamics and thus for testing the available theoretical approaches in extremely strong electromagnetic fields. In fact, this has already been successfully demonstrated in experiments performed for cooling conditions at the electron cooler of the ESR storage ring at GSI [1–4]. In one of the experiments performed for the zero average relative electron–ion energy [4], the recombination into the K- and L-shells was studied and it was shown that even for such extremely low-energy collisions the relativistic effects are important for proper interpretation of the measured data. Similarly, the first state-selective x-ray RR experiment performed at the ESR for different relative energies revealed an asymmetry of the measured K-shell rates with respect to the zero average electron velocity corresponding to the electron cooling condition. These results could not be explained [5] within the nonrelativistic RR calculations.
Here we report on the calculations of both nonrelativistic [6] and fully relativistic [7] K-shell differential RR rate coefficients performed for experimental conditions of the RR experiment carried out at the ESR [5]: namely, for bare uranium ions with an energy of 23 MeV u−1, recombining with cooling electrons characterized by transverse and longitudinal electron temperatures T⊥ = 120 meV and T∥ = 0.1 meV, respectively. The calculations were made for very low relative electron–ion energies, in the range from 0–1000 meV. In this experiment [5], the RR rate coefficients were measured by observing x-ray photons at small angles in the forward and backward directions with respect to the electron cooler axis. Consequently, the intensities of measured x-rays were very sensitive to fine details of the angular distribution of RR photons emitted, in particular, close to θLAB = 0° and 180° angles. Since the influence of relativistic effects on the angular distribution of RR photons is much stronger than on angle-integrated total rates, the relativistic calculations of the differential RR rate coefficients are important for proper interpretation of this experiment.
2. Theory
The RR rate coefficients are derived by convolution of the angle-differential RR cross sections dσRR/dΩ with the distribution of electron velocities in the electron cooler [8–10]:

The asymmetric Maxwellian distribution of electron velocity f(vrel,v) in the electron cooler, centered at relative velocity vrel, appearing in this formula, is expressed as follows [11]:
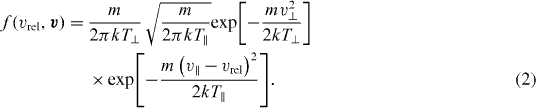
In order to obtain the angle-differential RR cross section, we applied two theoretical approaches, namely the nonrelativistic dipole approximation in the low-energy regime, which is expected to be well suited for low-energy electrons in the electron cooler [6, 12], and fully relativistic calculations [7].
In the low-energy regime (E ≪ Enl), i.e. when the electron kinetic energy E is much smaller than the electron binding energy Enl in the final state, the angular dependence of the RR cross section is described by the following formula [6]:

where is the Legendre polynomial of second order, ϑ is the angle between electron and photon momenta, and the asymmetry parameter βnl is constant for a given nl-state. In particular, for the K-shell β10 = 2 [6] and in this case the angular distribution of the emitted K-RR photons is described by the dipole term sin2ϑ.
For calculations of RR cross section for a given n-state, one can use the Bethe–Salpeter [13] formula (see also [14]), which, as a function of electron energy E in the ion frame, has the following form:

where σ0 = 2.1 × 10−22 cm2, is the hydrogenic binding energy of the electron in the K-shell of the H-like atom having nuclear charge Z and
is the Rydberg constant. Since the classical Bethe–Salpeter formula is valid only for high n-states, the so-called Gaunt factor Gn is introduced in equation (4) to account for deviation of this cross section from the 'exact' (quantum) result for low n-states.
The fully relativistic calculations of RR cross sections were performed within the framework of Dirac theory. Within this approach, the angle-differential RR cross section reads [15, 16]

where α is the fine structure constant, m is the electron mass, λc is the Compton wavelength and γ = (1 − β2)−1/2 is the Lorentz factor with β = v/c denoting the reduced electron velocity. In this formula, ψnbκbμb and ψpms functions are exact solutions of the relativistic Dirac's equation for the bound and continuum electron, respectively. The transition operator αuλ e−ikr describes the relativistic electron–photon interaction, which accounts, in addition to the electric dipole, for nondipole electric and magnetic contributions. The QED corrections to the RR cross section for capture into the n = 1 state of bare uranium are of the order of 1% [17] and were neglected in the calculations.
3. Results
As mentioned before, in the nonrelativistic dipole approximation the differential cross section dσRR/dΩ of photons for RR into the K-shell follows the dipole pattern sin2ϑ. Consequently, for the flattened Maxwellian distribution of electron velocities in the electron cooler (in the ion frame, under cooling conditions, the electrons are moving mainly perpendicular to the electron cooler axis) the distribution of RR photons in the ion frame has forward–backward symmetry. As can be seen in figure 1, this results in the symmetric nonrelativistic differential RR rate coefficient with respect to the zero average electron velocity.
Figure 1. Comparison of nonrelativistic [6, 12] and relativistic [7] differential K-shell RR (K-RR) rate coefficients plotted versus the relative electron–ion kinetic energy. Negative energy represents the case when electrons are moving slower than ions (vrel < 0).
Download figure:
Standard image High-resolution imageIn contrast, in the relativistic framework, the spin-flip transition, which is absent within the nonrelativistic dipole approximation [12], leads to an asymmetry in the recombination rate coefficient αRR(θLAB,vrel). When the electrons are moving faster than the ions (vrel > 0), the electron–ion collision axis, on average, coincides with the beam direction and, hence, K-RR photons are more likely to be emitted in backward directions in the laboratory frame, whereas the forward emission is predominant for vrel < 0. This can be seen in figure 2 where the relativistic total and differential K-shell RR rate coefficients calculated for different observation angles θLAB, plotted versus the relative electron–ion kinetic energy, are compared. This figure also shows that the total RR rate coefficients for the K-shell are much less sensitive to the relativistic effects than the differential rate coefficients calculated for the forward and backward directions in the laboratory frame.
Figure 2. Comparison of the relativistic [7] total αK-RR and differential αK-RR(θLAB) K-shell RR rate coefficients calculated for different observation angles θLAB plotted versus the relative electron–ion kinetic energy.
Download figure:
Standard image High-resolution image4. Conclusions
By performing systematic calculations of nonrelativistic and relativistic differential K-shell RR rate coefficients, we show that measurements of the electron energy dependence of the RR rates form a very sensitive tool for probing the fine details of the relativistic effects in RR at very low energies. We demonstrate that the relativistic effects lead to an asymmetry of the K-RR differential RR rate coefficients with respect to the zero relative electron–ion energy (cooling conditions).
Acknowledgments
This work was supported by the Polish Ministry of Science and Higher Education (grant no. N N202 463539) and the Helmholtz Alliance Program of the Helmholtz Association (contract no. HA216/EMMI).