ABSTRACT
A segregation between cluster members and field stars in the region of the double cluster h and χ Persei is accomplished working from proper motions and positions as kinematic random variables. The extinction RV and the distance moduli are determined once the physical cluster members are found.
Export citation and abstract BibTeX RIS
1. INTRODUCTION
The double cluster h and χ Persei has been the subject of a very extensive analysis through the years (Gahm & Arkling 1970), and an early work on position measurements was produced by Lamonth as early as 1869 (Alter, Ruprecht, & Vanyseck 1970, pp. 784 and 806). The two Galactic clusters form a double system (Oosterhoff 1937), and Struve was (Struve 1927) the first one to suggest that the general high concentration of early stars in the nuclei of the double cluster may constitute a grouping in space (Wildey 1964). Indirect evidence for the physical connection between h and χ Per was given by Pismis in 1953 (Tapia, Roth, & Navarro 1984). They are a pair of rich open clusters located at l = 13463, b = -3
72 and l = 135
08, b = -3
60, with the following equatorial coordinates for the B1950.0 epoch: α = 2h15
5, δ = +56°55' and α = 2h18
9, δ = +56°53' (Alter et al. 1970; Wildey 1964).
The h grouping has also been called NGC 869, Mellote 13 (Mel 13), Raab 9 (Rb 9), and Collinder 24 (Cr 24); χ Per has been named NGC 884, Mel 14, Rb 10, and Cr 25 (Alter et al. 1970). The investigations of many authors based mainly on astrophysical data show that the h and χ Per clusters are surrounded by the association Perseus I, rich in hot giants and in an extensive number of blue and red supergiants (Masevich 1957). These facts give a central role to the association Perseus I and to the double cluster in studies of stellar evolution.
The double cluster in Perseus is very well documented in the literature. Oosterhoff (1937) and Van Maanen (1920, 1944) made an extensive photographic study; Bidelman (1943), Johnson & Morgan (1955), and Schild (1965, 1966) determined spectral types for cluster members; Johnson & Morgan (1955), Wildey (1964), Schild (1965), and Moffat & Vogt (1974) performed optical photometry in various systems, and Mendoza (1967) and Tapia et al. (1984) worked on infrared photometry for the clusters. Recent photometric work was done by Denoyelle et al. (1990) and by Waelkens et al. (1990); a study of chemical composition is found in Dufton et al. (1988), and a catalog of star positions for three different recent epochs is found in Abad & García (1995).
The clusters are situated close to the Galactic equator in a Milky Way region of normal star density. This fact introduces a serious complication in the study of the physical properties of these clusters because the relative number of foreground and background stars is consequently very large. The proper motions are of much importance because they may help us to distinguish the physical members of the clusters from the optical ones (Oosterhoff 1937). In 1990 it was discovered that at least one‐half of the brighter stars in h and χ Per are variable stars and that most of these variables are Be or related objects (Waelkens et al. 1990).
There is no agreement on the age and distance moduli of both clusters. On the basis of a photometric study, Crawford, Glaspey, & Perry (1970) concluded that both clusters have nearly the same age and a distance modulus (m−M)0 close to 11.4 ± 0.4. Balona (Balona & Shobrook 1984) adopted a distance modulus of 11.16 for the double cluster, taking evolutionary effects into account. According to Schild, the distances to h and χ Per are about 2150 and 2500 pc, respectively (Schild 1967). Sometimes it has been assumed that h Per is younger than χ Per on the basis of the occurrence of B0.5 stars in h Per, while the earliest type stars in χ Per have a spectral classification of type B1 (Tapia et al. 1984).
The Hertzsprung‐Russell diagram of the double cluster differs greatly from the diagram of the surrounding association. These differences have a very definite evolutionary significance (Masevich 1957), and an accurate color‐magnitude diagram for the brightest stars of the double cluster shows that the reddening is not uniform. A comparison of Hertzsprung‐Russell diagrams leads to the conclusion that the nucleus and the association are about the same age but the formation of stars in the association continued after it had ceased in the nucleus (Masevich 1957). Other groups of stars in the Galaxy are known to be as young, but none so far investigated offers the possibility of such a large approximately homogeneous sample for study as the double cluster, together with its neighboring stars where early spectral type O5–B0 stars are found (Wildey 1964). Surely this has motivated the very recent and preliminary work by Selesnick and Massey on this cluster (Selesnick & Massey 1999).
The cluster χ Per is surrounded by supergiants five million years older than other groups of supergiants belonging to the h Per cluster and to an outer group of Perseus I centered between h and χ Per (Schild 1967). The association is seen projected on the Perseus arm at around 3500 pc. This arm contains late‐type supergiants, and consequently the probability that some of the supergiants attributed to the h and χ Per association are background stars is nonnegligible (Gahm & Arkling 1970).
Proper motions, positions, and radial velocities are the main membership criteria (Gahm & Arkling 1970). We now solve the membership problem in the region of h and χ Per considered as a double cluster, working from positions and proper motions obtained by Lavdovski (1961, 1965). Then the extinction RV, using the Johnson extinction method (Johnson 1965), and distance moduli are found.
2. THE DATA
Lavdovski published a catalog of proper motions and positions in the region of the double cluster h and χ Per (Lavdovski 1961). His publication also covers eight other open clusters and their vicinities: NGC 129, NGC 457, NGC 581, NGC 752, NGC 1912, NGC 2168, NGC 6885, and NGC 7092. The proper motions were determined by the film‐to‐film method using 32 pairs of plates taken with the Pulkovo normal astrograph. For the h and χ Per clusters, the plates are of very good quality; the epoch difference is 58 yr; the worked field has 128 reference stars and covers an 80' × 80' region with 2820 stars in the inner part, and a total of 3014 stars if those located in the outer region are considered (Lavdovski 1961). The research that he had begun in 1961 on the Galactic clusters NGC 869 and NGC 884 was completed in a later study where he published what he considered sure and probable members of the h and χ Per double cluster (Lavdovski 1965); he gives the Lavdoski and the Oosterhoff star identification numbers and membership determination made by Van Maanen in 1944 and Dieckvoss in 1956 (Oosterhoff 1937; Van Maanen 1944; Dieckvoss 1956).
Proper‐motion and UBV photometry work for 5504 stars in the region of the double cluster h and χ Per is also found in Muminov (1982).1 This is a photometric study and one of the latest reported works assigning new proper‐motion values in the region of the double cluster. He used two pairs of plates, though certainly with a greater epoch difference of 80 yr. Our work is based on the data of 2820 Lavdovski stars of the inner region coming from three pairs of plates of very good quality and with annual probable proper‐motion errors smaller than 00016 (Lavdovski 1961, 1965).
3. MEMBERSHIP PROBABILITIES WORKING FROM PROPER MOTIONS AND POSITIONS
3.1. Proper‐Motion Model
Let us define the Γ cluster,
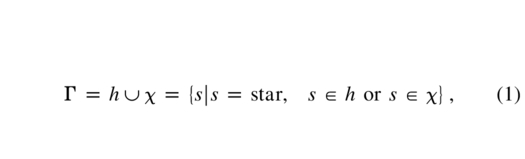
as a mathematical union of the h and χ cluster members.
The segregation between the Γ members and the field stars is carried out working from proper motions as random variables and on the basis of a mixed or contagious bivariate density function model obtained by overlapping two normal bivariate density functions, an elliptic one for the field stars and a circular one for the Γ cluster stars. It was first constructed by Vasilevskis, Klemola, & Preston (1958) and has been widely used in astronomical works. The vector point diagram (VPD) of proper‐motion data of a considered sky region where a cluster is supposed to be usually suggests its use, as it is suggested by Figure 1, a bidimensional plot of the proper motions of 2820 Lavdovski stars of the considered region (Lavdovski 1965). Thus, the model is given by
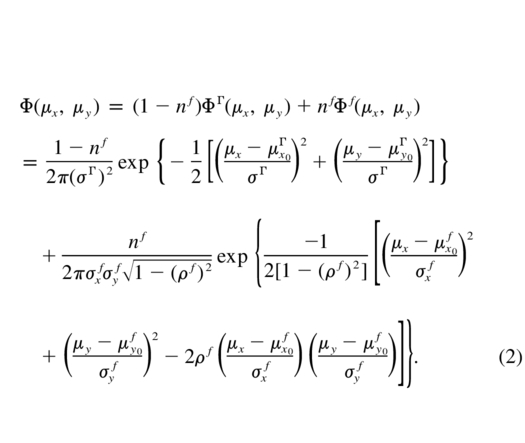
Fig. 1.— Decennial arcsecond proper‐motion VPD of 2820 stars in the region of the double cluster h and χ Per.
In this equation, μΓx0, μΓy0, μfx0, and μfy0 are the x‐ and y‐components of the centroids of the cluster Γ and of the bivariate model for the field distribution; σΓ, σfx, and σfy are the standard deviations of the cluster Γ and of the field star distributions; nf, nΓ, are the percentage of field and cluster Γ stars; 1 - nf = nΓ is the proportion of cluster Γ members; and ρf is the correlation coefficient of the normal bivariate field distribution. The mixed density function Φ(μx, μy) satisfies the relation
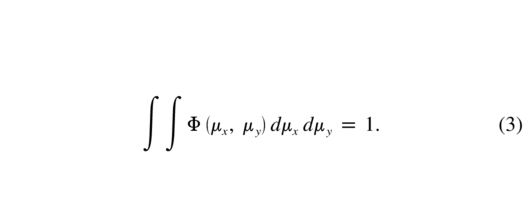
3.2. Maximum Likelihood Equations and Estimated Parameters
The parameters of the model are the Θ‐vector components,
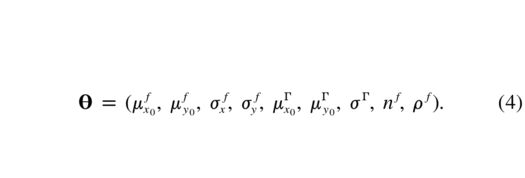
They are estimated by the maximum likelihood method, finding and solving a nine nonlinear equation system, where each likelihood equation has been derived in association with the unknown parameters of the Θ‐components. Following that order, the likelihood equations found are given by
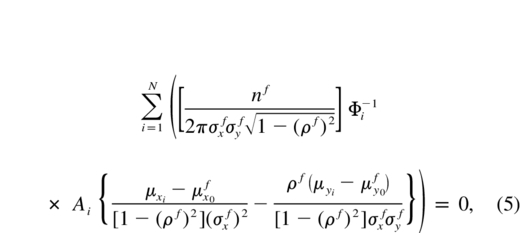
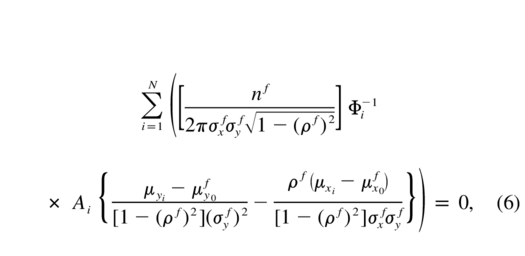
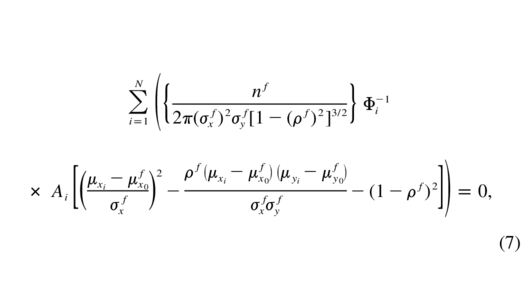
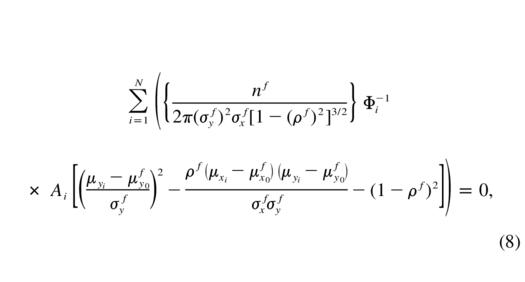
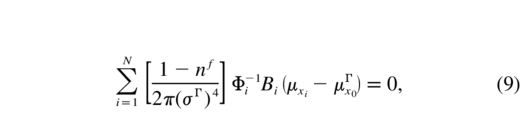
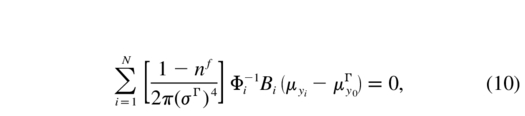
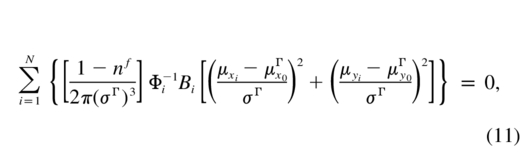
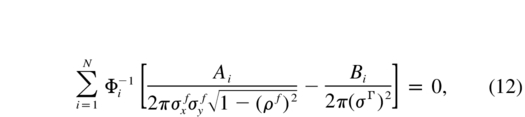
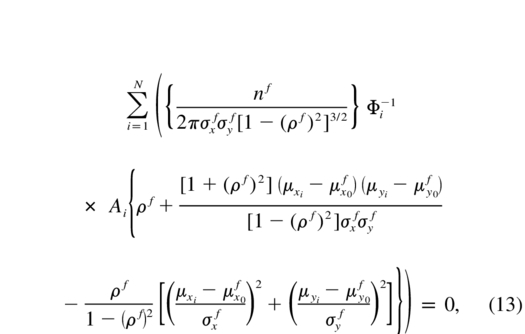
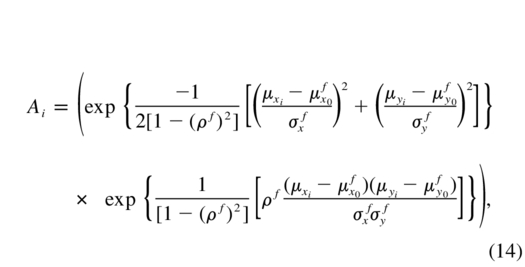
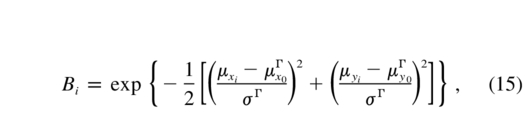
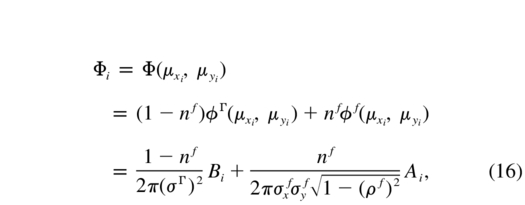
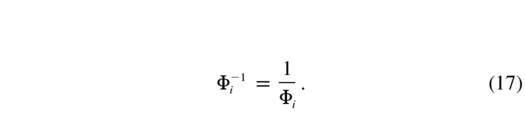
The estimated values for the parameters given in equation (4) are given by 0.026029, −0.026360, 0.100782, 0.073712, −0.006968, 0.009988, 0.024924, 0.190390, and −0.157710.
We have improved the appearance of the nine likelihood equations given them in terms of Φ−1. It is worthwhile to underline that the previous equations were obtained following a previous approach, without eliminating the correlation coefficient ρf (Brieva & Uribe 1990). The eight equations currently used by other authors who perform a rotation to a preferential reference frame can be obtained by making the correlation coefficient ρf equal zero (Sanders 1971; Slovak 1977; King 1983; Latypov 1978; Zhao et al. 1990). The equation system was solved numerically using the ZSYSTM routine, assigning initial parameter values as a starting point. This routine is an IMSL FORTRAN code that solves a system of nonlinear equations. It implements the Brown method of solution (Hiebert 1982), a modification of Newton's method that tries to improve on some aspects concerned with efficiency, uses Gauss elimination, and brings the solution of the nonlinear system; its main advantage is local quadratic convergence.
Although we have certainly experienced that the method is somehow sensible to variations of the starting parameters' estimations, only unreasonable initial values cause divergence. Therefore, we can claim that a local maximum for the likelihood function is found close to the automatic starting point we used (Sabogal‐Martínez et al. 2001).
In a general way, for each star with proper‐motion components μxi, μyi, the probability of being a cluster member is given by the Bayes rule,
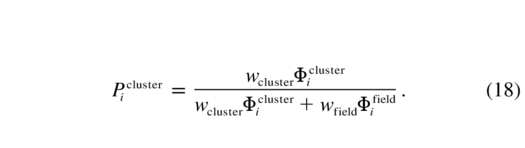
The symbols wcluster and wfield stand for cluster and field weights, respectively. From these probabilities the stars in the region may be ascribed to the field or to the Γ cluster using a suitable decision rule. The most usual but not the only one considers star i to be a cluster member if Pclusteri≥0.50, according to a Bayes minimum error rate of misclassification (Cabrera & Alfaro 1990).
3.3. Membership Probabilities Working from Position
Probabilities of being an h or a χ Per cluster member are assigned in a second step working now from position data. Once we know the members of the cluster Γ, the idea of giving greater membership probabilities according to position to the stars closer to the two nuclei of the region is followed. An exploratory data analysis of stellar positions suggested that bivariate Gaussian distributions be used to help purify the sample and to soften the general valid idea that results may be biased by the assumed shapes of the distributions of stellar positions.
In this way, the probability that the star i is an h or a χ Per member, given that it belongs to the Γ cluster, is respectively given by
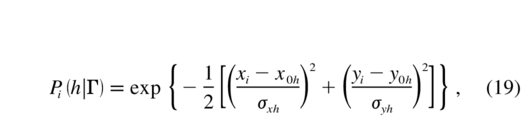
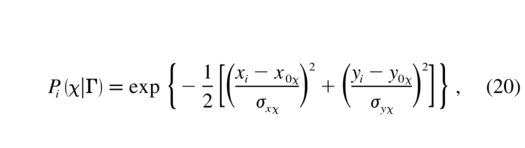
with x0h, y0h, x0χ, and y0χ, h and χ Per positional centroids; σxh, σyh, σxχ, and σyχ, star dispersions in the positional model.
The estimations of these parameters following the previous order are given by −0.15172900, −0.00179157, 0.11757300, 0.01163640, 0.14031700, 0.07121660, 0.16100800, and 0.09724440.
The Pi(h|Γ) and Pi(χ|Γ) membership probabilities are found for each star. In order to assign a star just to the h‐ or the χ‐component of the double cluster taking positions into account, the maximum probability value between Pi(h|Γ), Pi(χ|Γ) is chosen. In this way we have defined
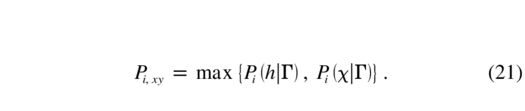
If Pi(h|Γ) is a maximum, the star is ascribed to h Per; if Pi(χ|Γ) is a maximum, the star is ascribed to χ. Once again, only those stars with probability values greater than 0.5 are considered to be physical members of h or χ. This procedure allows us to remove one cluster from the other working from positions.
3.4. Final h or χ Per Membership Probabilities
Let A and B, respectively, be the events: a star is an h or χ Per cluster member. Let C be the event: a star belongs to the Γ cluster. Applying a multiplication probability rule to the events A and C, a final probability value of being a member of h is given by
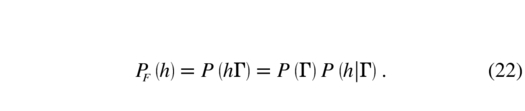
In a similar way, a multiplication rule taking the events B and C leads to a final probability value of being a χ cluster member
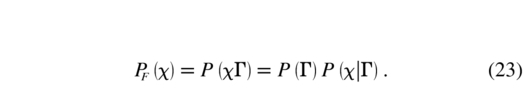
4. RESULTS
4.1. Membership Results
A piece of software called PROBADOUBLE.F was built. Using it we found 1554 members of the cluster Γ working from a catalog with the proper motions of 2820 stars; then h or χ membership probabilities according to position were also determined by equations (19), (20), and (21). In this way 558 stars were ascribed to h and 996 to χ. From equations (22) and (23), final membership probabilities were obtained with the following results (see Table 1), where PFi∶0.i≤P<0.i + 0.1), i = 0, ..., 9 are probability classes.
![]() |
The stars that we found as most probable members have been listed in Table 2. The meaning and contents of the columns are now explained. The first column gives the Oosterhoff identification numbers according to the information given in the Lavdovski catalog (Lavdovski 1961) and in the WEBDA database; the second column gives the star Lavdovski number; the third and fourth columns are the X and Y star positions; and the fifth and sixth columns give the μx and μy decennial proper motions. In column (7), we give our final membership probabilities (see Fig. 2). The membership probabilities assigned by Lavdovski to those stars that he considered either as probable (P) or as most probable (MP) members are given in column (8). In Figure 3, we have plotted the h and χ cluster members that we found (open squares and open circles, respectively). This figure allows a nice comparison between the stars that we found to be members and those stars considered by Lavdovski to be most probable (MP) or probable members (plus signs and minus signs, respectively). Although he used other membership sources and criteria (Lavdovski 1961; Van Maanenn 1944; Dieckvoss 1956), a high percentage of the stars considered members in the work of Lavdovski are also found to be members in our study working with the already explained procedure.
Fig. 2.— VPD of positions X and Y of the stars in h and χ Per. This figure discriminates among the stars according to our final probabilities; 344 stars with PF (h)≥0.5 are the most probable h Per members (open squares), and the 405 stars with PF (χ)≥0.5 are the most probable χ Per cluster members (open circles). The points stand for stars with low h or χ membership probabilities.
Fig. 3.— h and χ Per double cluster stars with membership probabilities greater than 0.50 according to our work. The open circles are the χ members that we found, and the squares are the h stars found in our work. The plus signs and minus signs are, respectively, the most probable members (MP) and probable members (P) according to Lavdovski.
![]() |
![]() |
![]() |
![]() |
![]() |
![]() |
![]() |
4.2. Extinction RV Determination, h and χ Distance Modulus, and Some Derived Results
In order to determine the extinction RV using the variable extinction method we apply the fundamental linear relation,
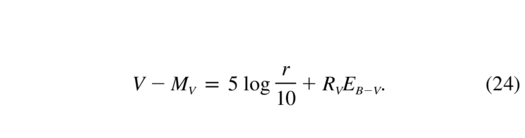
The most probable h and χ Per cluster members with published luminosity class, spectral type, color index B−V and U−B in the photometric Johnson system, and Henry Draper (HD), Bonner Durchmusterung (BD), and Oosterhof numbers allowing the identification of their Lavdovski numbers were found in the literature. Seven stars of the V luminosity class and another 10 with luminosity classes Ia, Ib, II, and III were identified; their data come from Johnson & Morgan (1955). For the former, MV was found using Blaauw's calibration and EB−V was obtained by an improved Q Johnson method, through the equation
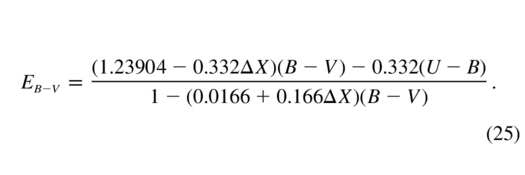
For the Ia, Ib, II, and III types, MV is from Blaauw, the intrinsic colors (B−V)0 come from Johnson (1958), and their color excess was found by

Figure 4 is a V−MV versus EB−V diagram where 17 points have been plotted. The slope of the fitted least‐squares regression line gives an estimate of RV, and the intercept gives an estimate of the double cluster distance modulus (DM), 5log (r/10). For each one of the variables involved in these calculations, let us say f(X1, ..., Xn), the estimated measurement errors have been found by the usual procedure:
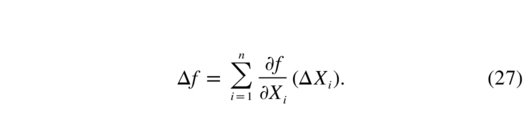
Fig. 4.— Stars used to determine the RV extinction. Their luminosity and probability classes are shown.
A mean extinction value A-V was found by

where E-B - V is an average value of the color excesses previously found.
The mean unit distance extinction a-V and the optical thickness τ-V come from the equations
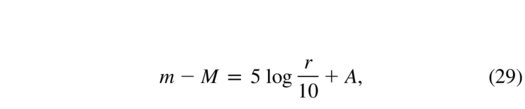
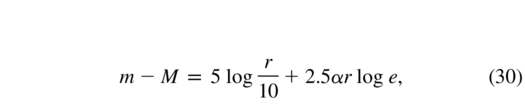
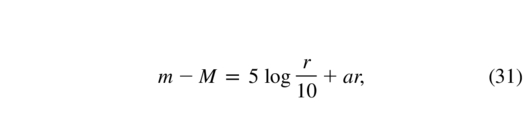
where a = 2.5αrlog e gives, in magnitudes, the unit distance extinction and
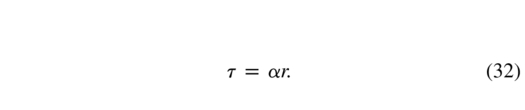
The color excess EU−V was calculated for each V‐type star by the Johnson method (Johnson 1958) and for the Ia, Ib, II, and III types by
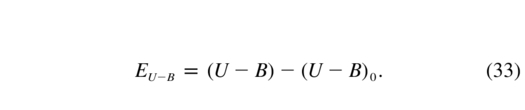
Finally, an average opacity in the visual, αV, comes from equations (30) and (31). All these results have been gathered in Table 3.
![]() |
4.3. Results of the Double‐Cluster Hertzsprung‐Russell Diagram
The photometric data for 39 members were obtained. The spectral types of 17 of them of luminosity class V are from Johnson & Morgan (1955), and (B−V)0 was found by an improved Q Johnson method. The (B−V)0 intrinsic color for eight stars of luminosity class V was found through the Q method by the equation
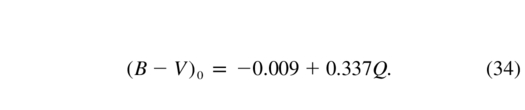
The intrinsic colors for 14 other stars of different luminosity classes were found in the Johnson intrinsic colors table of early‐type stars (Johnson 1958).
The magnitude corrected by extinction V0 was also obtained by the equation
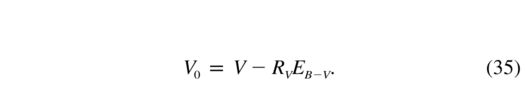
We also included the data of 12 M spectral type giant stars from Johnson (Johnson & Morgan 1955) with information about (B−V)0 and MV. Three of them are members, and for the other nine there were not proper motions. With these 51 stars we obtained a color‐magnitude diagram; then, on the basis of 19 nonevolved main‐sequence stars, 13 of χ and six of h, the following cluster distance moduli were found through a zero‐age main‐sequence (ZAMS) fitting (Blaaw 1963): 11.42 ± 0.09 (1953 ± 80) pc for h and 11.61 ± 0.06 (2099 ± 58) pc for χ and an average h and χ double cluster distance modulus given by 11.52 ± 0.05 (2014 ± 46) pc. These results are in very good agreement with recent results derived from a ZAMS fitting made by Marco & Bernabeu (2001): 11.66 ± 0.20 for h and 11.56 ± 0.20 for χ. Johnson's average distance is 11.7 ± 0.1 or 2188 pc (Johnson 1957).
Tangential velocity values given by 16.29 ± 0.1 and 16.67 ± 0.10 km s−1 for h and χ come from the equation
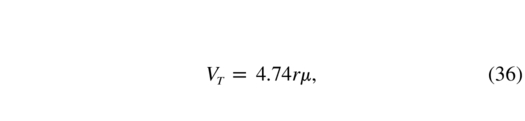
where the proper‐motion centroids (−0.007, 0.016) and (−0.007, 0.015) were found from membership results, using the proper motions of the 344 and 405 stars, members of h and χ, respectively. The radial velocity values of these two clusters have been published (Rastorguev et al. 1999); they are −53 ± 6 km s−1 for h and −40.5 ± 2.8 km s−1 for χ. Taking into account that these two clusters belong to the same Galactic region, the previous radial and tangential velocity values imply some disruptive tendency in the Galactic object called double cluster in Perseus.
Their MV absolute magnitudes were finally determined from the distance modulus, and an MV versus (B−V)0 plot was obtained. This double cluster Hertzsprung‐Russell diagram is shown in Figure 5. Johnson & Morgan luminosity classes (Johnson & Morgan 1955) and WEBDA types are given; the ZAMS of Blaaw and the main‐sequence overlapping are observed, and giants and supergiants are differentiated. The Hertzsprung gap is clearly established, and there is also a clear main‐sequence turnoff between the −4 and −5 mag MV values.
Fig. 5.— h and χ Per double cluster Hertzsprung‐Russell diagram. The ZAMS of Blaaw and the h and χ Per main sequence are shown.
5. DISCUSSION AND CONCLUSIONS
- 1.We contribute with an original and nonreferenced method to solve the membership problem in a double cluster. This a difficult problem to tackle; the cluster is situated closed to the Galactic equator, and the number of foreground and background stars is consequently very large; both clusters overlap extensively (Muminov 1982). Among the authors that have made a membership analysis of this cluster in the past are Oosterhoff, working from photometric data in 1937 (Oosterhoff 1937); Van Maanen, from proper motions in 1944 but without using the 1958 Vasilevskis model and the 1971 Sanders likelihood equations (Van Maanen 1944; Vasilevskis et al. 1958; Sanders 1971); and Muminov, working from proper motions and some photometric criteria (Muminov 1982).
- 2.Our procedure for solving the membership problem in the double cluster in Perseus working from proper motions and positions led to good results. The mixture density model for the proper motions of both the double cluster stars and the field stars, once the parameters were estimated by the maximum likelihood method, allowed us to split 2820 stars located in the cluster region into 1554 members of the double cluster and 344 field stars; 996 were pruned. Then, working from position data and through a multiplicative probability rule, final membership probabilities were found; a minimum error Bayes rate of misclassification allows us to consider as most probable members those with final calculated probabilities greater than 0.50. We found 344 most probable members of h and 405 most probable members of χ, in good agreement with Lavdovski membership results using other membership sources and criteria (Lavdovski 1961; Van Maanen 1944; Dieckvoss 1956).
- 3.We point out that the mixture density model and the likelihood equations that we used working with proper motions as random variables are also an original contribution. The maximum equations were obtained without eliminating the correlation coefficient ρ and have a different algebraic formulation from the one we used in a previously published paper (Uribe & Brieva 1994). These nine likelihood equations can be reduced to the eight equations currently used by other authors, who perform a rotation to a preferential reference frame by making the correlation coefficient ρ equal zero (Zhao et al. 1982).
- 4.The suggested procedure for splitting the double cluster into h and χ members worked correctly and could be used to solve the membership problem in other Galactic double clusters.
- 5.From the color index B−V, spectral type, and luminosity class of 17 most probable members of the double cluster and using the improved Q Johnson method (Johnson 1958) and the absolute magnitude calibration given by Blaauw (1963), we found a value for the total‐to‐selective extinction ratio RV, fitting a linear least‐squares regression line to 17 points in the diagram, color excess EB−V versus distance modulus V−MV. The slope of the fitted straight line was 3.20 ± 0.04; it allowed us to determine the extinction RV. The intercept gave an estimated distance modulus for the physical members of the double cluster; its value was 11.84 ± 0.04 mag. From RV and the color excess EB−V, a mean extinction in the visual band A-V, equal to 1.71 ± 0.07 mag, was found. Some other important physical quantities leading to valuable knowledge about the extinction phenomenon produced by the interstellar Galactic dust between the Sun and the double cluster region, strongly affecting the stars' electromagnetic radiation, were also found: an average value for the color excess E-B - V, the mean unit distance extinction a-V, the optical thickness τ-V, and the average opacity in the visual AV.
- 6.The tangential velocities that we found and radial velocity values published by other authors suggest a disruptive tendency in the Galactic object called the Perseus double cluster.
- 7.On the basis of the 3.2 RV extinction value in the direction of the double cluster and RV values equal to 6.6 in the I Ara direction (Johnson & Morgan 1955) and 5.7 toward the Orion Sword region (Johnson & Morgan 1955), we observed that the dispersion and absorption of the electromagnetic radiation coming from the double cluster is small in comparison with the higher values found for the other mentioned regions. Besides, the value 3.2 ± 0.04 that we found for RV is in good agreement with the value 3.0 ± 0.1 found by Johnson in 1965 (Johnson 1965); the small difference may be explained by the fact that we worked with physical cluster members obtained from proper motions and positions. Some other authors did not link the membership problem with a previous determination of RV. Cramer got 2.75 ± 0.01 in 1982 (Cramer 1982). The distance modulus DM = 5log (r/10) found by Johnson was 11.7 ± 0.1 (Johnson 1957); the average color excess E-B - V value, 0.56 ± 0.01, found by Johnson in 1957 (Johnson 1957) was also independently obtained in 1990 by a research group from the Geneva Observatory and Belgium (Waelkens et al. 1990). An average extinction A-V = 1.85 ± 0.12 was found by a Mexican research group in 1984 (Tapia et al. 1984).
- 8.A Hertzsprung‐Russell diagram was built, and distance moduli were found for h, χ, and h+χ through a ZAMS fitting (Blaaw 1963) working with 19 nonevolved main‐sequence stars, 13 of χ and six of h. A small disagreement with Johnson may be explained by the fact that our results rest on a previous membership analysis and the fact that few members with enough of the required photometric and spectroscopic data were found in the literature. Johnson's distance modulus for the double cluster was 11.7 ± 0.1; we obtained 11.52 ± 0.05 mag, a mean value between the distance moduli of 11.42 ± 0.09 for h and 11.61 ± 0.06 mag for χ. It must be taken into account that the apparent magnitudes V and the color indexes B−V were previously corrected by extinction before the construction of the Hertzsprung‐Russell diagram. The distance modulus 11.84 ± 0.04 found using the extinction results of this work is larger than either 11.42 ± 0.09 or 11.61 ± 0.06, the h and χ distance modulus values derived through the ZAMS fittings. This may be explained by the different number of main‐sequence stars used to fit the regression line in the former case (Fig. 4) and those used to fit regression curves to the h and χ nonevolved main sequences.
- 9.Surely, an improvement in the found results would come from more photometric and spectroscopic data for the cluster members.
Since the very beginning of our work in open clusters some years ago, we have received advice through letters, private communications, and computing assistance. That is why we are very grateful to Kyle Cudworth, Mark Slovak, Jean‐Claude Mermilliod, William Van Altena, Jack MacConnell, Emilio Alfaro, Terry Girard, Mario Lattanzi, Guiseppe Massone, Alessandro Spagna, Marco Missana, and the deceased Stanislaus Vasilevskis and Natale Missana. We also want to acknowledge the Division of Research of Universidad Nacional de Colombia DIB, for continuous support given to the Research Proyect 803471 and to COLCIENCIAS. We thank the galactic group of the Observatorio Astronomico and mainly Alejandro Garcia and Beatriz Sabogal, whose work in the theses leading to their physics degrees supports some of the achievements of this paper.
Footnotes
- 1
Muminov (1982) is also available on the Web at http://adc.gsfc.nasa.gov/adc/authors.