Abstract
The QCD multipole expansion (QCDME) is based on quantum field theory and has been extensively applied to study transitions among ϒ and ψ family members. As it refers to non-perturbative QCD, however, it has only a certain application range. Even though it successfully explains the transition data among members of the ϒ (ψ) family, as Eichten indicates, beyond the production threshold of mediate states it fails to match data by several orders of magnitude. In this work, by studying a simple decay mode D*→ D + π0, where a pion may be emitted before D* transitions into D, we analyze the contribution of QCD multipole expansion. As the Dπ portal is open, the dominant contribution is an OZI-allowed process where a light quark-pair is excited out from vacuum, and its contribution can be evaluated by the 3P0 model. Since direct pion emission is OZI-suppressed and violates isospin conservation, its contribution must be much smaller than the dominant one. By a careful calculation, we estimate that the QCDME contribution should be 3–4 orders smaller than the dominant contribution and this result can offer a quantitative interpretation for Eichten's statement.
Export citation and abstract BibTeX RIS

Content from this work may be used under the terms of the Creative Commons Attribution 3.0 licence. Any further distribution of this work must maintain attribution to the author(s) and the title of the work, journal citation and DOI.
1. Introduction
The QCD multipole expansion (QCDME) has been widely used to calculate transition rates among heavy quarkonia by pion emission [1, 2]. Since this theory refers to non-perturbative QCD, it has a limited application range, beyond which the theory is no longer applicable. When the masses of the charmonia (bottomonia) are sufficiently larger than the production thresholds of D(*) D̅(*)(B(*) B̅(*)), which may become on-shell intermediate states, as Eichtein et al. indicate, the decay widths evaluated in terms of the QCDME are smaller than the data by three orders of magnitude [3]. In other words, the dominant modes of, say, ϒ(nS)→ ϒ(mS) + π+π− or ϒ(nS)→ ϒ(mS) + π0 can be realized via ϒ(nS)→ B(*) B̅(*)→ ϒ(mS) + π+π−, which is usually referred to as the final state interaction or re-scattering process. Even though the re-scattering process dominates the transition, there can exist an additional direct pion emission to the dominant mechanism which can be evaluated in terms of the QCDME.
It is interesting to theoretically estimate how small the contribution of the direct pion emission could be in comparison with the dominant contribution. For this, we adopt a simple decay mode, and calculate the contribution of direct π0 emission to the decay rate of D*→ D + π0, since D*→ D + ππ, which does not violate isospin, is forbidden by the phase space.
The direct π0 emission occurs before the system transitions into the final D-meson. The π0 is produced by the gluon fields originating from either the charm quark or the light anti-quark. The emitted gluon fields are not real gluons but gluon fields which may be EN or MN modes with parity assignments being (−1)l and (−1)l+1 respectively. For phenomenological studies, one usually just takes the lowest order contributions in the expansion according to the parity requirement; because the pion emission occurs via the strong interaction, parity must be conserved. In this decay mode, π0 is a pseudoscalar meson with isospin 1, thus the direct one-pion emission is OZI (Okubo-Zweig-Iizuka)-suppressed and violates isospin conservation, even though the decay mode is neither OZI-suppressed nor isospin violating. The isospin violation is due to the anomaly triangle where md − mu is not zero. Later we will show that the leading contribution can be perfectly described by the 3P0 model and the results are consistent with the data. Looking at the contribution from direct pion emission in comparison with the dominant contribution, however, our numerical results show that the effective coupling constant gD*Dπ determined by the QCDME is 60–70 times smaller than that obtained from quark pair creation (QPC).
In Section 2, we evaluate the contributions to the decay rate of D* + → D+π0 from both the quark pair creation (QPC) described by the 3P0 model and the direct pion emission described by the QCDME in Subsections 2.1 and 2.2 respectively. The numerical results are presented and comparisons with the corresponding experimental data are made. In the final section we discuss the framework in some detail and then draw our conclusions.
2. D*+ → D+π0
2.1. The quark pair creation model and its application to π0 radiation
In the framework of the QPC model [4–21], the decay D* + → D+π0 occurs via a quark-antiquark pair creation from the vacuum. It is an OZI-allowed process. The decay mechanism is displayed graphically in Fig. 1. Many soft gluons are emitted from the quark and anti-quark legs, which then annihilate into a quark-antiquark pair. Equivalently, the physics scenario can be described as a quark pair being excited out from the vacuum. The 3P0 model has been widely applied to calculate such hadronic strong decays.
Fig. 1. Quark-pair creation from vacuum serves as the decay mechanism for D*+ → D+ π.
Download figure:
Standard image High-resolution imageThis process has been calculated by some authors [22] where they only gave the numerical results but not the details of the calculations. We have repeated the calculation and obtained a rate which is consistent with theirs. For readers' convenience, we have collected the relevant formulations about the calculation in terms of the 3P0 model in Appendix A. The transition operator for the quark pair creation reads
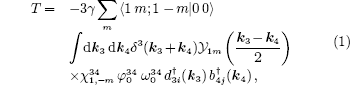
and the hadronic matrix element is determined as

In Eq. 1, i and j are the SU(3)-color indices of the created quark and anti-quark respectively. are for flavor and color singlets, respectively.
is the spin wave function.
is the ℓth solid harmonic polynomial. γ is a dimensionless constant which denotes the strength of quark pair creation from vacuum and is fixed by fitting data. Following Ref. [23], we take γ = 13.4 in this work. For Eq. 2, the explicit expressions for the wave function of a meson and the hadronic matrix elements are presented in Appendix A.
The helicity amplitude of this process can be extracted from the relation
. Then, the decay width corresponding to the process is written in terms of the helicity amplitude as
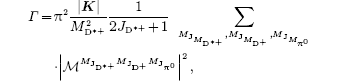
where we take KD+ = −Kπ0 = K in the center of mass frame of the D*+.
Numerically, we take a typical R value for the D meson from Ref. [17] as 2.3 GeV−1 and R = 2.1 GeV−1 for π0 from Ref. [23]. With these parameters, the decay width of D*+ → D+π0 can easily be obtained and we have got it as 21.9 keV, which coincides with the result given in Ref. [22]. Experimentally, the decay width of this mode is 25.6 ± 1.0 keV [24]. The consistency of the numerical results evaluated in terms of the 3P0 model with data indicates that the theoretical framework is well established and applicable to describe such processes.
On the other hand, based on the heavy quark effective theory (HQET), one can extract the effective coupling constant of D*Dπ from the previously calculated decay width, which might offer significant information for application of an effective theory. Following Ref. [25], the related effective Lagrangian can be written as

Then we can get the decay width as

with . The transition amplitude of D*+ → D+π0 is [25]

where is the polarization vector of D*. From equation (4) we obtain gD*Dπ = 0.51.
2.2. The QCDME and contribution of direct π0 emission to the decay width
The pion can be directly emitted before D* transits into D, thus the amplitude, in principle, should be added to the process depicted in the above subsection and interferes with it. Just by the qualitative analysis, the one-pion emission is an OZI-suppressed process and moreover, it violates isospin conservation, therefore must be very small compared to the vacuum creation.
It is interesting to investigate such an effect in other processes, i.e. as long as the one-pion emission is not a leading term, how small it would be compared with the leading terms. Below, we will quantitatively investigate direct one-pion emission in D*→ D + π0.
The corresponding diagrams for direct pion emission in D*+ → D+π0 for which the QCDME is responsible are shown in Fig. 2.
Fig. 2. The QCDME diagrams responsible for pion emission in the process D*+ → D+π0.
Download figure:
Standard image High-resolution imageFig. 2 is just obtained by distorting Fig. 1, as shown in Fig. 3.
Fig. 3. Distortion of the 3P0 decay mechanism for D*+ → D+π into an OZI-suppressed process.
Download figure:
Standard image High-resolution imageHere we only draw two gluon field lines, but as well understood, in the QCDME scenario the lines correspond to a field of EN or MN mode, which are by no means free gluons, and the line also does not correspond to a single-gluon propagator. Thus the line denotes a collection of many soft gluons just as shown in Fig. 1.
Now let us calculate the rate contributed by the processes shown in Fig. 2 in the framework of QCDME.
The process of directly emitting a soft π0 from D* in the decay D*→ D+π0 is dominated by an E1 − M2 coalescence transition. This is an OZI-suppressed process and violates isospin conservation. The transition amplitude is [26]
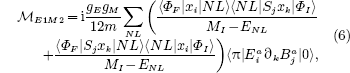
where the S operator acts on the total spin of the heavy-quark and light-anti-quark system, N and L are the principal quantum number and the orbital angular momentum respectively of the intermediate hybrid state, MI and ENL are the mass of the initial meson D* and the energy eigenvalues of the hybrid state respectively, and m is the energy scale of the M2 transition, which we set to be mc and in our numerical computations. The amplitude reduces to [27, 28]
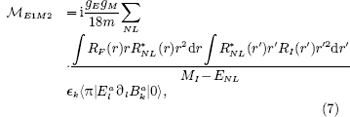
where is the polarization vector of D*, and RI, RF and RNL are the radial wave functions of the initial, final and intermediate hybrid state, respectively.
The radial wave functions are calculated by solving the relativistic Schrödinger equation [29]. The potentials for the initial and final D(*) mesons are taken from Ref. [29] and the potential for the intermediate hybrid states is taken from Ref. [30].
The matrix element is of the form [27, 28]

where gE and gM are the coupling constants for the color electric field and color magnetic field, and k is the momentum of π0.
In order to compare the results with the effective coupling constant gD*Dπ obtained by using the QPC model, the transition amplitude of D*+ → D+π0 can be rewritten as [25]

is the effective coupling constant obtained by means of QCDME, which is then
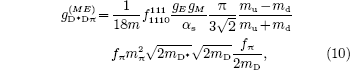
with
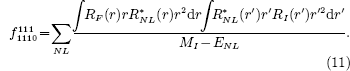
For our numerical analysis, the input parameters are taken from Ref. [24]. Here mD = 1.869 GeV, mD* = 2.010 GeV, mπ = 0.135 GeV, mc = 1.800 GeV, mu = 0.3 GeV, , fB* = 0.230GeV, fK = 0.160 GeV, Fπ = 0.093 GeV and
. αs = 0.31 for
. Following Ref. [1, 2, 27, 28] we set
,
with αE = 0.6 and for a possible error range, according to the literature, we let αM vary from αE to 10αE. The constants
and effective coupling constant g obtained in terms of QCDME are listed in Table 1.
Table 1. Coupling constant and
(in units of GeV−3), with αM set to be αE, 3αE, 10αE, and 30αE separately, and the value of m set to
.
αM = αE | αM = 3αE | αM = 10αE | αM = 30αE | |
---|---|---|---|---|
![]() |
5.677 | 9.833 | 17.952 | 31.094 |
![]() |
0.00145 | 0.00251 | 0.00459 | 0.00794 |
In order to explore possible validity ranges of QCDME, we increase the maximum value of αM to 30αE, whereupon reaches 0.00794. It is thought that the size of heavy quarkonia might be much smaller than 1/ΛQCD, whereas, for D(D*), because of the existence of a light quark those sizes may be larger. But some model dependent calculations show the mean square root radius R to be R = 1.94 GeV−1 for J/ψ in terms of the relativistic-like Schrödinger equation [29], whereas Kuang et al. obtained R = 2.13 GeV−1[28]. For the case of the D meson, R = 2.3 GeV−1, which is slightly larger than the expected value for J/ψ, but not by much, and it is obviously smaller than 1/ΛQCD ∼ 5 GeV−1. Therefore, we believe that the errors brought up in this scheme are tolerable. With possible errors, this result is 60 times smaller than that obtained by the QPC model [24, 31].
In Table 2 we list the decay widths Γ(D*+ → D+π0) from the QCDME contribution, and see that when αM takes the maximum value 30αE, the decay width of Γ(D*+ → D+π0) from the QCDME contribution is 5.31 × 10−3 keV. This result is more than three orders of magnitude smaller than the result obtained by using the QPC model(21.9 keV).
Table 2. Decay width of Γ(D*+ → D+π0) (in unit of keV) from QCDME contribution, for αM we take αE, 3αE, 10αE, and 30αE respectively.
αM = αE | αM = 3αE | αM = 10αE | αM = 30αE | |
---|---|---|---|---|
Γ(D*+ → D+π0) with ![]() |
4.43 × 10−5 | 5.31 × 10−4 | 4.44 × 10−4 | 5.31 × 10−3 |
Γ(D*+ → D+π0) with m = mc | 1.77 × 10−4 | 1.33 × 10−4 | 1.78 × 10−3 | 1.33 × 10−3 |
3. Discussion and conclusions
Our numerical results for the decay mode D*→ Dπ0 confirm that direct pion emission is not the leading term and the contributions determined by the QCDME must be much smaller than those induced by other mechanisms.
Let us try to understand the smallness of the contribution from direct pion emission, using data to show that our estimate is reasonable. There are two suppression factors: one-pion emission violates isospin conservation, and QCDME is an OZI-suppressed mechanism. Compared with vacuum quark pair creation, it must be small and we see that its contribution to the decay width is in the range of about 10−4 − 10−5 keV. The decay ψ(2S)→ J/ψ + π0 is also an isospin violating and OZI-suppressed process, and its branching ratio is 1.27 × 10−3 [24], slightly larger than our estimate for D*→ Dπ0. That further suppression factor comes from the fact that the transition ψ(2S)→ J/ψ + π0 is an S-wave process, whereas D*+ → D+π0 is a P-wave process whose decay width is proportional to the three-momentum k, which is small, so the decay width Γ(D*+ → D+π0) suffers from a P-wave suppression.
We may also look at ψ(2S) as an example for direct π emission. ψ(2S)→ π0 + hc(1P) can only occur via a direct pion emission, so is completely determined by the QCDME mechanism, and its partial width is about 0.26 keV. Since it is an S-wave process, it does not suffer from P-wave suppression. In ψ(2S) decays, the mode ψ(2S) → ηc + π0 is not seen, but ψ(2S) → ηc + π+π−π0 has been measured, and its branching ratio is not too small (< 1.0 × 10−3), because the direct emission of three pions does not violate isospin conservation.
Equivalently, the QCDME can be replaced by chiral perturbation theory, for example the transition of ϒ(nS)→ ϒ(mS) + π+π− has been studied in terms of the chiral theory [32].
By the discussion above, we conclude that for the decays of mesons containing a heavy quark and a light anti-quark, the contribution from QCDME is much smaller than that caused by the QPC mechanism, thus when evaluating the decay rates, one need only take into account the contribution from the QPC mechanism and the direct pion emission can be safely ignored.
In conclusion, we confirm the validity of the QCDME and determine its application region. Since the framework applies only to direct pion emission, if there are other mechanisms to contribute, such as quark-pair creation from vacuum to D*→ D+π0, or ϒ(5S)→ BB̅*→ ϒ(1S)+π+π− where an intermediate state of the BB̅* portal is open, i.e the available energy is above the production threshold of BB̅*, then the direct emission induced by the QCDME is no longer the leading term and can only contribute a tiny fraction.
We greatly benefitted from a talk given by Prof. Eichten at Nankai University and we also sincerely thank Prof. H.Y. Cheng for enlightening discussions.
:
Appendix A. Some formulae
In the 3P0 model, for a two-body decay process A → BC, the total wave function of a meson can be written as
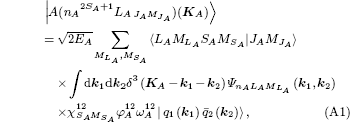
which satisfies the normalization condition: . The subscripts 1 and 2 refer to the quark and anti-quark within meson A. kA is the momentum of meson A.
,
,
are the spin, flavor and color parts respectively. ψnA LA MLA(k1,k2) is the spatial part of a meson wavefunction in the momentum representation. When the mesons concerned are in the ground states, the simple harmonic oscillator (HO) wavefunction is employed, which reads as

where k = (m2k1 − m1k2)/(m1 + m2) is the relative momentum between the quark 1 (with mass m1) and the anti-quark 2 (with mass m2) within a meson. The R value is fixed by fitting experimental data.
The explicit matrix element is depicted as
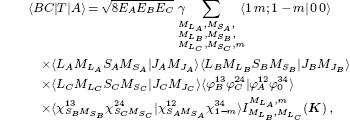
where is a spatial integral, reading as
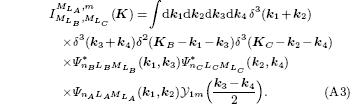
Footnotes
- *
Supported by National Natural Science Foundation of China (11375128)